02. Basic Transmission Genetics
EEB 122. Principles of Evolution, Ecology and Behavior

Lecture 02. Basic Transmission Genetics

https://oyc.yale.edu/ecology-and-evolutionary-biology/eeb-122/lecture-2

The genetic material is deoxyribose nucleic acid. We have known that since 1945, and we've known its structure since 1953. And this is actually an extremely important point: Genes are solid particles that are transmitted from parent to offspring. They are not fluid but actually?material stuff. They encode information, as sequences of nucleotides, and in the DNA it's adenine, thymine, guanine and cytosine. So you can think of those as four letters (ATGC). They string into a linear chain to form a molecule, and here are two strands that are twisted around each other to form a double helix.?The sugar phosphate strands form the backbone, and then the nucleotides are glued onto the backbone and they form pairs; so adenine pairs with thymine, and guanine pairs with cytosine.?
The sugar phosphate backbone is the same in every DNA molecule on the planet, and the information in the molecule is in the sequence of nucleotides. You can think of that as letters forming words. So these are big molecules. If you were to put all the chromosomes in your nuclei together, and just for one cell, and string them together, one haploid copy is exactly one meter long. So just chop this piece of measuring tape up into 26 pieces and you get about the size that you've got in each of your chromosomes. So we're not talking about tiny, weensy, little molecules. DNA is a biggie, and it's very stable.?

How does this relate to organisms? Well that's the issue of genotypes and phenotypes, and that's a question of information and matter. The genotype is basically the info in the DNA, and every cell in your body has got all the information in it that is needed to build a whole organism. If we can overcome some of the genetic programming of the oocyte, of the egg, we could, in principle, simply put a cotton swab into your cheek and take one cell off of your cheek and then do fancy reproductive medicine and clone you, off of just the DNA in a cheek cell. Now it turns out that the developmental machinery in the egg is really critical, and it's hard to do that. But just from the point of view of the information, any cell in your body could be used to make another you.?
The phenotype--basically you should think of that as you. That's the material organism which is?built according to genotypic instructions. So the genotype contains information, the phenotype contains matter, and the transformation from information into matter is done by developmental biology. Decoding that transformation is one of the major research agendas for the twenty-first century in biology. It's called the construction of the genotype/phenotype map, a?modern jargon for developmental biology.?
So where does the DNA actually sit in the cell? Well here's some more vocabulary.?
The eukaryotes, the things that have a real nucleus--which includes us and all other multi-cellular organisms, plus a whole bunch of single-celled ones--they have cells that have a nucleus and the DNA in the nucleus is contained in chromosomes. The DNA resides in multiple linear chromosomes in the cell nucleus.?And the DNA itself is actually wrapped around proteins in the chromosome.
In the prokaryotes, which are the things that lived on this planet for about the first two billion years of life--that is, bacteria and archaea--they are single-celled organisms, and their DNA is basically not in separate chromosomes, but all in one circular loop that is?attached to the cell?wall. The DNA forms a continuous loop, called a circular chromosome.
There's a big difference in the way that eukaryotes and prokaryotes are organized, and in fact the eukaryotic nucleus is very probably the evolutionary residue of a prokaryote; that's where that organelle probably came from.?The other organelles,?that used to be independent organisms, are?mitochondrion, chloroplast?and spindle apparatus (the spindle apparatus that pulls the chromosomes apart has a little circular genome associated with it).

The number of chromosomes is usually constant, within a species, although there is some variation. You've got 46 sitting in every cell of your body, 23?from your mom and 23 from your dad,?except your red blood cells which don't have a nucleus. That dual set, one from mom and one from dad, together it's called the diploid condition.?
And in contrast to that, your eggs and your sperm are haploid. So the gametes are haploid. They have one set. Haploid means one set of chromosomes. So the haploid number in humans is 23. The diploid number is 46. World record for a eukaryotic minimum chromosome number is 1. Ascaris, a nematode that lives in the gut of dogs, has 1 chromosome. World record for maximum number of chromosomes? Actually it's probably also in ascaris, but in the somatic condition. That one chromosome falls into about 1000 pieces when it develops. So chromosome number varies widely.?
You can think of a chromosome as being about 1000 genes,?a gene as having several thousand nucleotides in it,?and?a gene as being a segment of DNA that tells a cell to make a particular protein, a particular structural RNA, and through splicing and other things there are various other classes of?regulatory RNAs.
You're made out of proteins and materials whose construction is basically governed by the actions of proteins. And so the DNA in your genome is a set of instructions on how to make what kinds of proteins at certain places and times to control the construction of the organism and determine the uniqueness of the species.
Genes are in specific locations and they come in different forms.?We call the place that a gene is found on a chromosome its locus; this is in classical genetics.
Genes can be found in different versions. We call those different versions alleles. For example, the gene for eye color is either blue or brown. Those would be the allele for blue or the allele for brown.
If you are carrying two different versions of the gene--you got one from your mom and you got one from your dad and they're different--then you're a heterozygote, and we call that condition the heterozygous condition.
If you got the same one from both parents, then you're a homozygote, and we call that the homozygous condition.
Normally a gene has got a codon--that is, three nucleic acids--that say, "This is where you're going to start reading me off."
And then it's got another one down at the end that's a stop codon, that says "That's where you stop."
And then in between these two codons,?you've got a long string of DNA--this is in eukaryotes, not in prokaryotes--a long string of DNA, and some of it is going to end up coding for protein and some of it is not.?
The part that will code for protein we call the exons; the part that is going to be cut out and spliced and put into messenger RNA, to go out and make protein.
And the part that is not we call introns. So not all the DNA is going to go out and become protein.?

The central dogma of molecular biology, basically, is that DNA makes RNA makes protein. And transcription is copying the DNA into messenger RNA, and that's done with complementary pairing, and in the process, the thymine is replaced by uracil in the messenger RNA. The introns are cut out and discarded. The exons are spliced together and the RNA is then translated into protein in the ribosome (The RNA?is?spliced and?assembled?into?a molecule?that is then going?to code for a?polypeptide; or a big polypeptide?is?a protein.?And that will then go through a?ribosome?to make protein).?
Because of the amount of engagement of RNA in this very basic process of life that we think that RNA was probably the original genetic molecule, and that DNA evolved after RNA, and then all of this process developed after that.?The reason for that is that RNA has a very high mutation rate; DNA has a low mutation rate. But RNA can be an enzyme and DNA is not. So RNA was both an information storage molecule and an enzyme, at the beginning, close to the beginning, of life, and then DNA came along later.?
Transfer RNA is a much smaller molecule. And it is the molecule that matches the genetic code, that's sitting there in the messenger RNA, to a particular amino acid. And this whole process will get fed through a ribosome, and out at this end of it the amino acids will get joined together. So the RNA will go out one part of the ribosome, and out of another part will come the growing chain of the protein. It takes three nucleotides to specify one amino acid.?The DNA is a codon sequence. It gets translated into an RNA, and then in units of three or?in chunks of three nucleotides, the RNA gets translated into protein.?

Information is flowing out from the genotype into the phenotype. It doesn't go in the other direction. This is a re-statement of something that August Weismann said in the nineteenth century.?The implication of that is that evolution of acquired characteristics,?characteristics acquired during the lifetime of the parent,?won't work.
When the cells divide, the DNA replicates and each daughter cell gets a complete copy. This is how inheritance works. This is why you look like your parents. During replication the ends of the DNA strand are loosened and opened up so that in the notch between the two strands the nucleotides can be inserted. And all of this is done with complex enzymatic machinery and it's done extremely precisely.?
Only one mistake in about a billion nucleotides occurs in DNA. It's almost impossible for humans to construct a system that has that degree of reliability.?Natural selection has worked very hard to get those enzymes that precise. When a mistake does occur, that is one source of mutation. And, in fact, the more frequently DNA is copied, the higher the mutation rate. So that's one place where mutations come from.?
When this is going on, this copying is going on in the process of the development of?a?multi-cellular eukaryote, like yourselves, or when it's going on in an asexual, uh, eukaryote, basically what happens is the chromosomes go through the process of mitosis. And in mitosis,?what's going on is that the chromosomes will be duplicated, they will line up at a plate, at the center of the cell; spindles will form. So these are proteins, these react in the fibrils here, and they are anchored to an organizing center, which is at the poles of the cell, and they attach to the centromeres of the chromosomes, and they pull one copy into each cell, and then the cell splits.?

If you've got two genes, A and a, that are alleles at the same locus, the two versions of the gene at the same place on the chromosome.?
Mitosis basically consists of a doubling--They line up at the middle of the cell, and then the spindle apparatus pulls one copy of the A and one copy of the a?into each of the daughter cells.
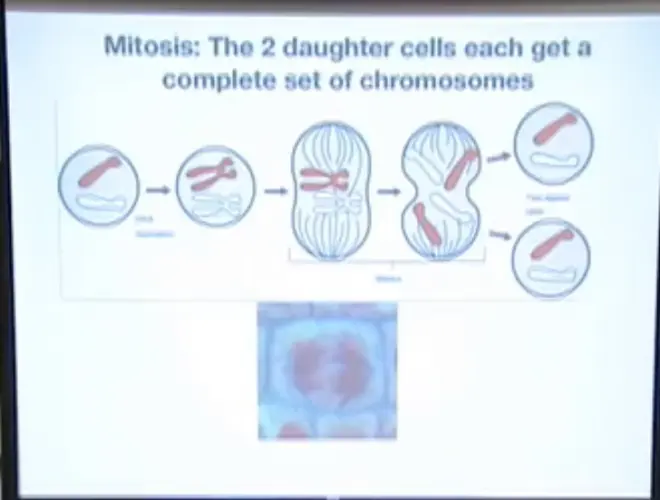
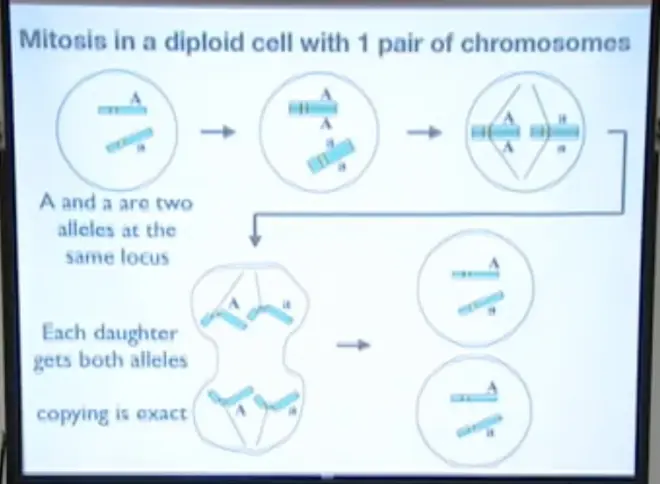
Meiosis is the process that produces gametes.?So it takes the diploid parent down into a haploid gamete. So it's a reduction division. The process is more complicated, and in fact it is like sticking two mitoses together in a sequence. The chromosomes are duplicated and they are then actually duplicated again. Then out of the original chromosome there are two?out of the original cell.?And as a result of that, each haploid gamete is getting one original chromosome, or the other, but not both.?
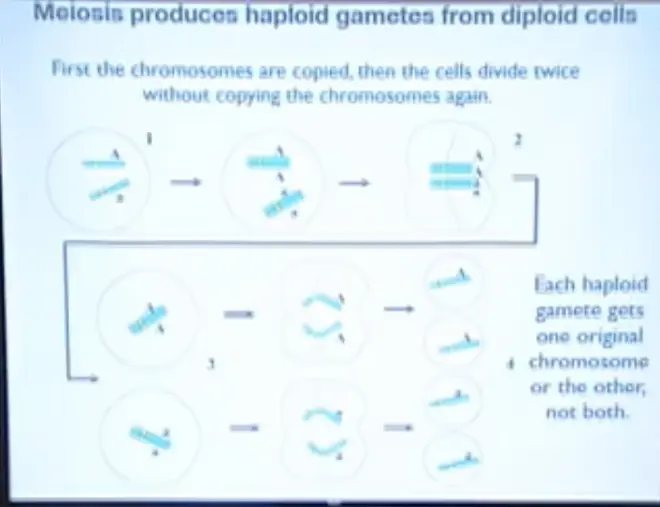
Does the behavior of chromosomes explain Mendel's Laws? And it does. So Mendel's First Law is that if you have two alleles, two members of a gene pair, when they segregate into the gametes, one goes into each gamete; that's Mendel's Law of Segregation.?
Half of the gametes from a heterozygous Aa, will carry the A allele, and half of them will have a little a allele.
If you have two heterozygotes who are mating with each other--so the male gametes have either A or a, and the female gametes have either A or a, it is Mendel's Law of Segregation which tells us that we can expect those gametes to be equally likely. The probability is 50% in each case. When they then come together to make a zygote that's going to grow up to be the offspring, then these--we just multiply these probabilities together. So .5 times .5 gives us .25, and each of these kinds of zygotes is equally likely; 25%.
If A is dominant, that is say it's brown eyes, and a is recessive, say it's blue eyes--and remember our baby with issues--then the ratio here is 3:1. That's only true because--it's 3:1 because in these three cases we have a A, and in this one case we don't. So the ratio is 3:1. Some genes are dominant and some genes are recessive. If a gene's dominant, you can see that fact in the phenotype; you can see that the allele is present in the phenotype. If it's recessive, you can't see the presence of the gene in the heterozygote. Its presence is covered up by the dominant one.
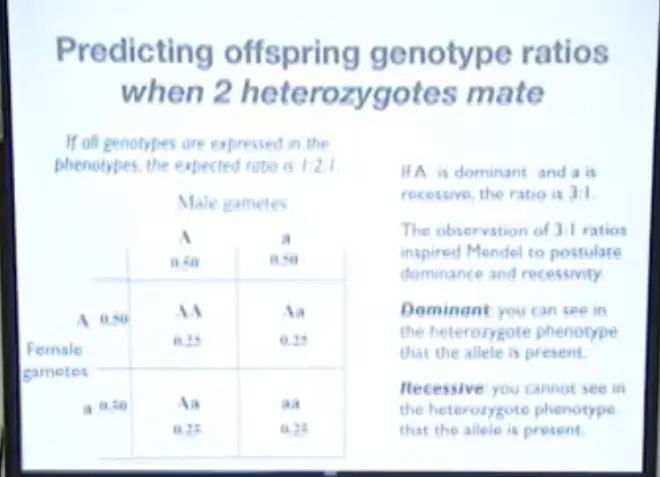
Mendel's Second Law: What happens when we're looking at two genes and they're on different chromosomes? Well Mendel's Second Law basically says that the events that occur at the different chromosomes are independent of each other. So genes that are sitting on one chromosome are going to be assorting independently to genes that are sitting on other chromosomes.
If we have Aa--and this would be a Aa heterozygote; this is a Bb heterozygote. They are depicted as already having been copied. So they've been duplicated so that they can start going through the process of meiosis. And what's going to happen is that we're going to pull them apart.
We're going to make four gametes out of each of the chromosomes. This combination, where you get AB and ab, is just as likely as this combination, where you get Ab and aB. So that's tracking what happens when you have genes on two different chromosomes that are forming gametes. That's Mendel's Second Law.?
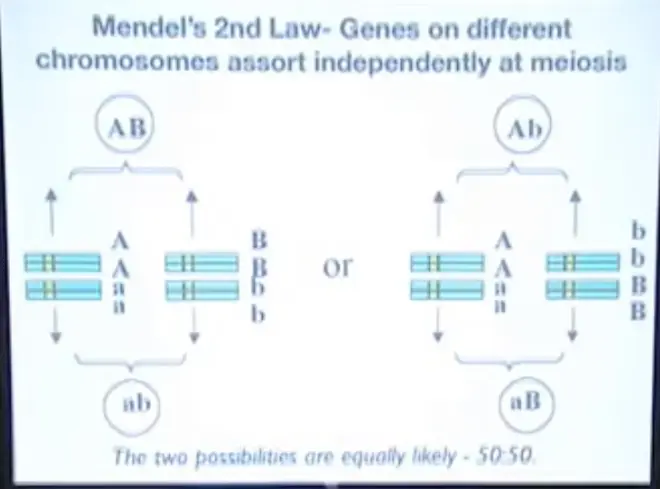

So meiosis is capable of producing genotypes that are different from the parental genotype.?The fact that the offspring gene--genotypes are different from the parental genotypes is the essential evolutionary fact about sex. It can be achieved in a lot of different ways, but it means that sex produces offspring that are not copies of the parent; they are all different from the parent. And there are two genetic mechanisms that do it.?
If you've got the genes on different chromosomes, they assort independently?(independent assortment). The chromosome assortment generates new combinations within the genome.
If they're on the same chromosome, you can have crossing over. Crossing over means that chromosome parts are exchanged during meiosis, and it produces new combinations.?The chromosomes can break and be rejoined, and the product of that is gametes that are different. These are recombinant gametes generated by crossing over.?The crossing over generates new combinations?within chromosomes.
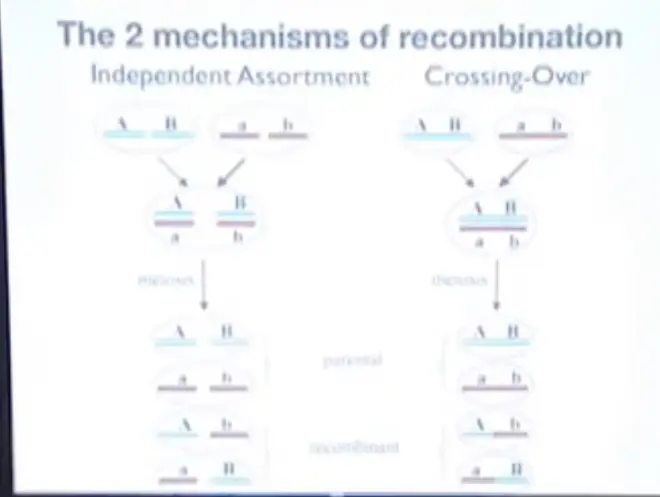
These combinations, this kind of genetic variation, is something that's going on in every generation. It is thought that every human chromosome experiences one crossing-over event every generation, roughly; probably true for most organisms.?

It's mutations that occur in the germline--that is, in the cells that will form eggs and sperm--that get transmitted to offspring. They have evolutionary significance. So they change the information that's transmitted over evolutionary time.?
Mutations that occur in somatic cells are things that lead to cancer. Cancer is a mutational process, and every cancer is a little evolutionary process that occurs just within the lifetime of the person who has it.?Ultimately?through the history of life, mutations are where all genetic variation came from.?
We refer, on the one hand, to point mutations. That's where you just change one nucleotide, and there's a category--there are categories of point mutations. You can have substitutions and?deletion.?
A deletion of an entire codon will not cause a change in the downstream amino acids.?
If you take out three nucleotides at once, there won't be any change in the coding for the remaining amino acids.
If you take out one or two, you're shifting the reading frame. So if you have a deletion of one nucleotide or two nucleotides,it changes everything downstream, from that point. So one or two deletions can have really big effects on the information content of the whole genome. We call those frameshift mutations.
Mutations also occur at higher levels. You can have chromosomal mutations where you delete entire genes, therefore?taking out maybe 3000 nucleotides. And the whole gene disappears.
These are very important evolutionary processes. If you duplicate a gene, you can use the old copy to keep things working while you innovate with a new copy. So gene duplications are really important.?

It turns out that genetics and random mating,?the whole structure of the Hardy-Weinberg assumptions, is set up in such a way that information is preserved at the level of the population. That makes evolution possible. If we didn't have that retention of information, then you couldn't tweak it; it would get eroded by processes other than natural selection.?So it's kind of an inheritance mechanism at the whole population level. It minimizes conflicts among genes about who gets into the next generation.?
Jill and John have this baby, and the baby is at issue. Should he be worried?
Jill is a recessive homozygote. She's got two copies of a.
John could be either a dominant homozygote, or he could be a heterozygote; he's got brown eyes. The baby's got blue eyes, and is a recessive homozygote.
John's genotype is a random sample of those on the island, and therefore that q2--that's the frequency of aa--is 0.01. So if q2?is 0.01,?what is q? .1. Right; 10% probability.
The?probability that John is a heterozygote is 2pq.
The probability that John is a dominant homozygote is p2; p is .9; p2?is .81; 81% probability that John is a homozygote.?
The only way that that baby could be John's child is if he is a heterozygote. 2pq is 18%; p2?is 81%.?
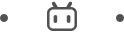