14. Coriolis Force and Storms
GG 140: The Atmosphere, the Ocean, and Environmental Change

Lecture 14.?Coriolis Force and Storms

https://oyc.yale.edu/geology-and-geophysics/gg-140/lecture-14

Pressure Anomalies on Weather Maps [00:00:00]?
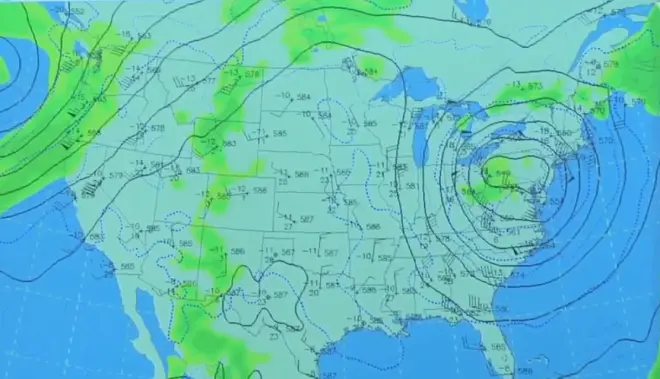
This is the 500 millibar map, so when data is analyzed above the Earth's surface, it's normally done on constant pressure surfaces. It's not exactly constant altitude, but it's more or less a flat, horizontal surface. And what's contoured in black is actually the altitude itself. So that constant pressure surface is a little bit rolling. But the thing to understand is that the level of constant altitude on a pressure surface is very much analogous to an isobaric curve on a level surface. So you can think of these as isobars, these heavy black lines. The temperature--lines of constant temperature, the isotherms, are in the dashed blue lines.
The data from which it is all derived are these little clusters. To the right of the symbol is the altitude of the 500 millibar surface with one zero left off. So at that station, the 500 millibar surface is 5,870 meters above sea level. The temperature is 27 Celsius, and the dew point is minus 11 Celsius.?The winds are given too in a little wind barb using the convention of a short feather being five knots, a long feather being 10 knots, and a thick feather like that being 50 knots.
So we're talking about geostrophic balance. If you look at these values--549--so this is a low-pressure center, so this would be called a cyclone. It's not at the Earth's surface, necessarily. It's about five kilometers up in the atmosphere. But that would be a cyclone. So higher pressure around it, lower pressure in the center.?The winds go counterclockwise around that because we're in the Northern Hemisphere. And notice how well the winds parallel the isobars. And so these conclusions have followed from the geostrophic balance assumption. And the closer the isobars are together, the stronger the wind will be.
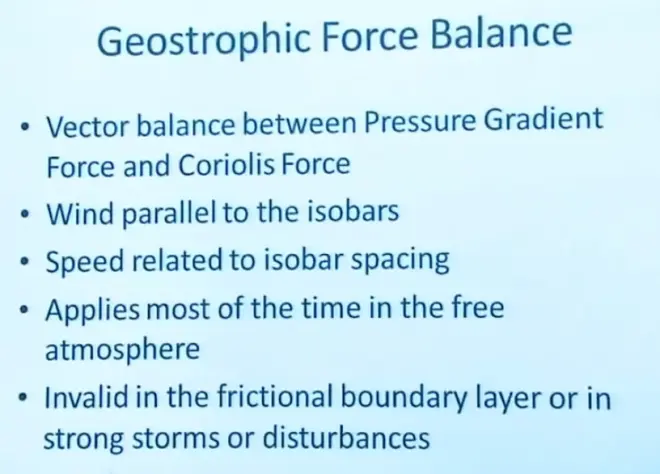
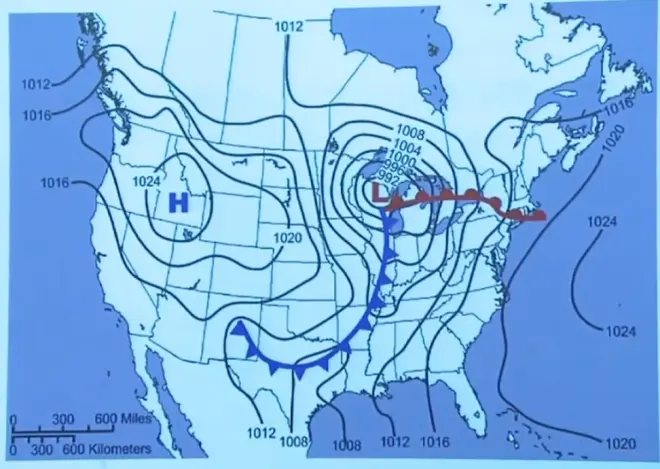
This is?an example of an isobaric map at the surface of the Earth. These values are sea-level pressure, so wherever the pressure was measured, it was reduced to a common altitude, sea level, and then the contours were laid on it.?
And here we had an anticyclone and a cyclone. The only problem is that this is down close to the surface of the Earth, and in some cases even below the surface of the Earth.?When you're right down near the surface of the Earth, there is some friction added to the winds as well, and that will break the geostrophic constraint maybe not too badly, but it'll break it a little bit. What tends to happen when you're near the surface is that the winds, instead of blowing right along the isobars, tend to rotate in a little bit.?That subject is called the?Ekman layer,?a description of how those winds turn in the boundary layer.?
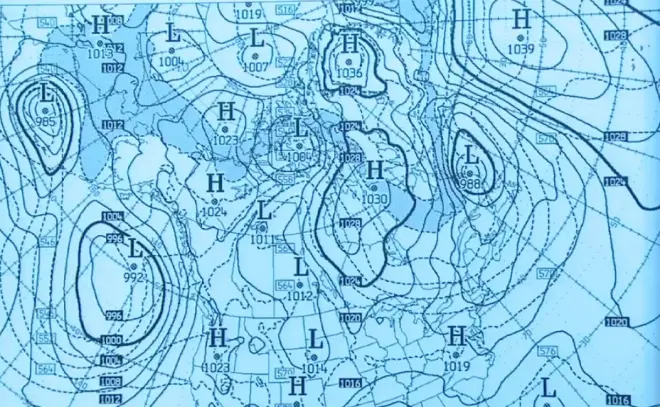
If you expand out to look at most of the Northern Hemisphere, you can see that at any given time, there are lots of highs and lows, cyclones and anticyclones. And again, no wind is given on this, but you can get a pretty good idea of what the wind is.?And the speed of the wind is related to the packing of the isobars.?So the mid-latitude and high-latitude atmosphere is just loaded with these eddies. And one of their jobs is to pump heat northward to balance the heat budget of the high latitudes and low latitudes.?
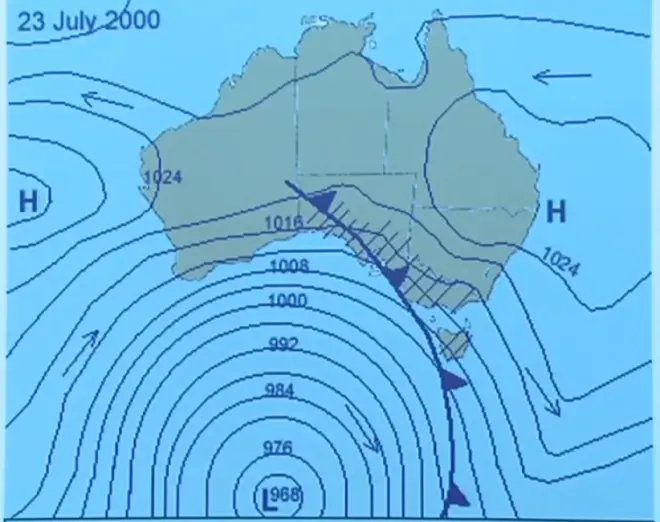
If you go to the Southern Hemisphere--here's Australia--of course, now everything is backwards, because the Coriolis force is reversed in the Southern Hemisphere. It acts to the left of the motion vector. And so those relationships that we derived have to be reversed also.?Here is a low-pressure center. We would call it a cyclone. And they've indicated the arrow here indicating the direction of circulation, and indeed, it is clockwise.?
Geostrophic Adjustment [00:09:57]
First of all, geostrophic adjustment. It's fine to claim that the Earth's atmosphere is in geostrophic balance most of the time. But I think an even more fundamental question is how does it come to be in geostrophic balance? And that process is called geostrophic adjustment.
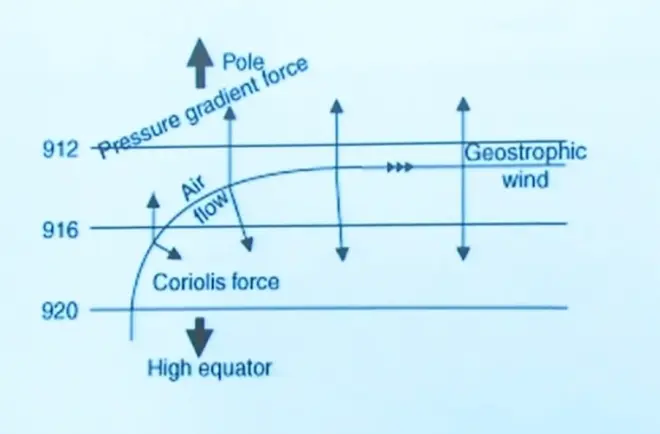
Let's assume at the beginning moment that you've got a parcel of air sitting there not moving. Well, you know that's not in geostrophic balance, because it's in a region that has a pressure gradient. So it's going to have a pressure gradient force. But if it's not moving, it can't have a Coriolis force, so those two forces cannot possibly be in balance.
So here's your parcel not moving. I release it, and it's going to immediately feel the pressure gradient force pushing it towards the north. It's going to start to accelerate in that direction. So as the minutes and hours tick by, starting with zero speed, it's going to have then maybe one meter per second, two meters per second, three meters per second.
But now, as it picks up speed, it's going to start to feel a Coriolis force, small at first, because remember, the Coriolis force is proportional to the wind speed. So after some time, it may have moved to here, and it will have developed a little bit of Coriolis force, and it'll start to turn. The Coriolis force is a deflecting force. It'll start to turn.
Throughout all this time, however, because I've drawn these isobars equally spaced, the pressure gradient force on that parcel will remain the same. So it's always being pushed to the north by the pressure gradient force, but as it moves and accelerates, it turns and accelerates and eventually ends up moving east with a speed that will give it an equal and opposite Coriolis force and pressure gradient. So that will--that process of geostrophic adjustment usually takes about 12 hours in the atmosphere.
Parcels could be disturbed by getting caught up in a thunderstorm. They could be disturbed by running against a mountain range and being deflected, running against a building and getting deflected. But then, as the hours pass, it naturally tends to want to return to a state of geostrophic balance. It's a natural tendency in the atmosphere for the wind to try to readjust itself to geostrophic balance.
The same thing happens in the oceans.?The Coriolis force acts on ocean currents, and ocean currents are even in a more rigorous state of geostrophic balance than is the atmosphere. So these same principles we're learning now apply to ocean currents as well.
You might ask what would happen if a hurricane tried to cross the Equator? Well, would it have to switch its direction of rotation? The answer is probably so, but it's never been observed to happen. A hurricane has never successfully crossed the Equator. In other words, it's constructed itself to be appropriate with the sense of the Coriolis force in the Northern Hemisphere. If you tried to push that into the Southern Hemisphere, it would have to really fall apart and then rebuild itself in the opposite direction.
Coriolis Force and Toilet Bowl Mythology [00:18:23]
I was going to talk about toilet bowl mythology. The legend is that if you flush the toilet bowl in Australia, the water will swirl down in the opposite direction to that in the Northern Hemisphere.
Well, I don't think that's true. The problem is that the Coriolis force acting on a small scale is a rather small force, and other things may dominate. And here's what would dominate in the case of a toilet bowl or any small body of water. If you take a tank of water and fill it and you're even the least little bit careless, you're going to be pouring the water into that off-center a little bit, and you're going to be giving it a little bit of swirl to begin with, just from the way you filled the basin.
Now, when you pull a plug at the bottom and that water begins to move towards the center and then drain out, the conservation of angular momentum will amplify whatever little swirl is in there. And so it'll end up swirling faster and faster as you draw the water out, but in the direction of the swirl that you gave it initially. And in almost every case, that's going to dominate over that weak Coriolis force acting on such a small scale.
Now, this idea was tested some years ago up at the Woods Hole Oceanographic Institution. They took a large tank of water, about this size and about my height, and they filled it ever so carefully to avoid swirl.?They then lowered into it a kind of a honeycomb set of dividers that, once it was down in there, would stop any swirl that was existing. They then pulled it out carefully and waited for several days to get rid of any other remaining swirl.
Then they pulled the plug in the middle at the bottom. And it took several hours to see it, but gradually, there was a swirl observed, and it was due to the Coriolis force. In other words, as the air moved—as the water moved in towards the center, the deflecting force took the water that way, this one took the water that way, acting to the right of that motion towards the center,?they did get a cyclonic swirl, or?a counterclockwise swirl?due to the Coriolis force.?But I tell that story because of the extent you have to go to to get that effect to dominate over the initial swirl. So don't expect to find that as you switch hemispheres.?
Definition of a Storm [00:24:51]
We're going to start talking about storm types.?The tough thing is to define a storm.?A?meteorologist's definition would be that it represents some kind of a local energy transformation, a transformation from one kind of an energy to another, for example, maybe potential energy to wind energy, to kinetic energy of motion, or something like that.
It's not a physical object. In fact, the air that's involved in a storm might even be flowing through it. The air that's in a storm today, that storm may still exist tomorrow, but it may have a whole different set of air that comprises it. So it's not a physical object. It's a pattern of winds, of vertical motions of clouds, of precipitation in the air. They're patterns of complicated fluid motion within a larger atmosphere.
Now, they may be dangerous, but I don't want to define it that way. I don't want to define a storm as something that necessarily causes damage or takes human lives. In fact, in some cases, it may be the only thing that brings needed rain. Most types of storms produce precipitation in some way, and in many climates, that is the rain producer. So storms should not be considered all bad.
Typically, they are short-lived, however. I can't think of any examples where a storm of any of the types I will define in just a moment exists more than three or four days. Now, there may be other storms nearby that grow as that one decays, but if you try to track an individual storm, like a hurricane, normally, a few days, and they've gone through their life cycle. Some of these have natural life cycles, and some of them just die for other reasons.
And I've indicated that we're interested in climate in this course as well. But you can't define climate without understanding storms either. Because the storms that occur in a particular location are part of the definition of the climate. For example, here in New Haven, we get a certain amount of rain in the summertime, and almost all of that comes from thunderstorms. We get rain in the wintertime, and almost all of that comes from mid-latitude frontal cyclones. So without knowing something about storm types, you really don't have much to say about climate either. They really are part of the recurring patterns of temperature and precipitation and wind that occur in any particular location.
Convective and Frontal Storms [00:29:26]
Now, with those definitions, I want to break them down into two broad categories, convective and frontal. And convective storms are those that are driven by latent heat release. We're going to talk about two or three different kinds of convective storms, but they all share the property that the energy that drives them is the heat that's released when water vapor condenses to form liquid or ice. And because they depend on latent heat release, you need to have a lot of water vapor to begin with in the air, and that means these are going to be warm-season storms--warm air can hold more water vapor--or storms that occur in the tropics, where you have lots of water vapor all the time.
But anyway, they're warm storms. You're not going to get thunderstorms in--very rarely would you get a thunderstorm in high latitudes, up in the arctic, for example. It's not unheard of, but it's quite rare. And when you do get it, it's only because there's been a big push of warm air temporarily up into the high latitudes that has allowed a thunderstorm to occur there.
A frontal storm, the other broad category, their energy source is the horizontal gradient in temperature. If I have cold air next to warm air, that represents a potential source of energy because the warm air would like to rise, and the cold air would like to sink. So holding them side by side is only a temporary thing. When I release it in some way, motion is going to begin to occur, and in some cases, that'll develop into a frontal storm.
I say cold season because, remember, due to the tilt of the Earth, it's in the wintertime in mid-latitudes when you've got the big differential heating. The pole is getting no sunlight, the Equator is getting a lot, and so it's in the cold season of each year for the respective hemispheres that you get the strong temperature differences, which will then allow frontal storms to form.?Now, this is easy to remember, because the definition of a front itself is a region of strong temperature gradient. So it's easy to remember what is the energy source for a frontal storm.
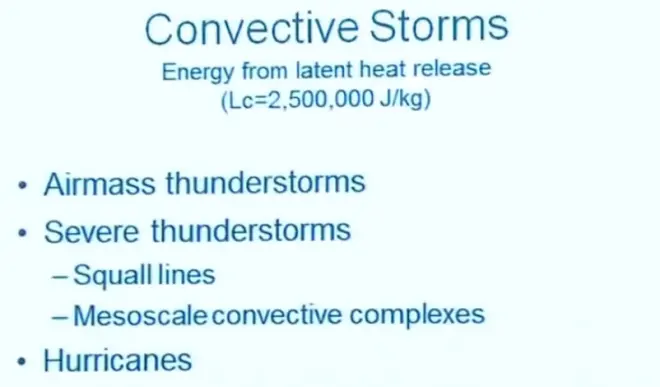
So first, we're going to talk about convective storms. And I'm just going to talk about three. Airmass thunderstorms, severe thunderstorms?and hurricanes.?I'm not going to say much about the different types of severe thunderstorms, but there are subcategories within the general domain of severe thunderstorms.?
So what I'm saying is that all of these storms are driven by the energy from latent heat release. And remember, that number is about 2.5 million joules per kilogram of water vapor. So for every kilogram of water vapor that you condense, you get 2.5 million joules of energy added to the atmosphere in the form of heat. But then that may make that air rise, and that'll get the whole storm going. So it all goes back to this rather large latent heat that's present when water vapor condenses.
Airmass Thunderstorms [00:33:24]
So the first and the most benign of these is the airmass thunderstorm. It's called an airmass thunderstorm because basically there's a property of the atmosphere that would allow them to form. And so when we say that a warm, tropical air mass has moved into New England with the properties of being warm, not very stable, and not very strongly sheared, then thunderstorms can occur. So we've kind of included here the fact that it's the nature of the air mass that is going to control whether or not these thunderstorms occur. And that's why they're called airmass thunderstorms.
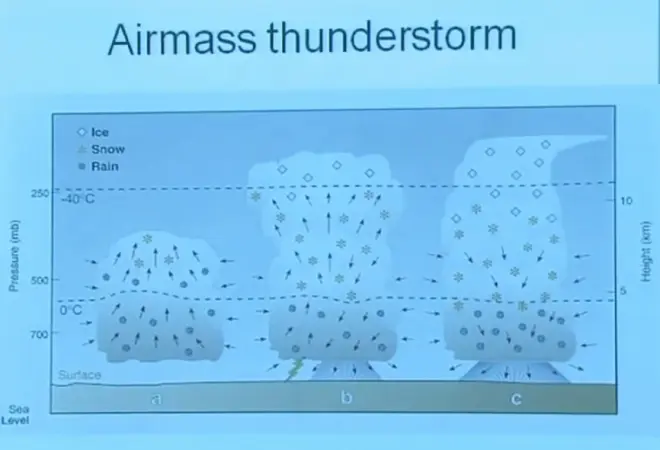
So they go through a life cycle. They start as a fair-weather cumulus cloud, but there is enough water vapor in the atmosphere so that as the air begins to rise, the latent heat addition is sufficient to keep that air moving upwards. It'll keep going, under many circumstances, all the way until it reaches the tropopause. It'll flatten out at the tropopause, maybe overshoot it a little bit, but generally flatten out.
And about the time it gets there, it'll start to rain heavily out the bottom. The process by which the raindrops are made is almost always the ice phase mechanism in these clouds. Remember, it may be very warm at the surface--after all, this is a summertime phenomenon--but nevertheless, because it gets colder as you go up--and here's the zero degrees Celsius line--once that cloud gets above the zero degrees Celsius line, you've got supercooled water that'll then start the ice phase process going to generate at first snowflakes. Then they'll melt as they fall, and they'll fall as rain out the bottom.
There may be some lightning coming out of those clouds. If they are moving rapidly across the landscape, they will just give you a brief shower, which won't be too much of a problem. If they happen to be stationary, even though they may only last for an hour, if they're stationary, they might cause some local flooding. So whether they cause flooding depends not only on the rain rate from these systems but how fast they're moving across the landscape, whether they're distributing that rain as they move.
There might also be some--of course, the lightning can cause damage. You could be struck by lightning, or it could cause a fire. But normally, these are not so damaging. In fact, they bring us most of the summertime rainfall that we count on in New Haven and most of the rest of the world. In the warm season or the warm climate zones, this is the primary rain producer. So it's definitely a positive thing.
The air that is rising is generally converging into this storm until the rain begins.?Once the rain begins, the rain falls below cloud base into air that has a relative humidity less than 100%, and some fraction of the raindrop?water mass is going to evaporate before those raindrops hit the surface.?That evaporation causes some cooling in this area. And once that is well established, the cooling by evaporation of raindrops, that cool air begins to fall to the surface and spread out. When it does that, it shuts off the inflow. Here we needed inflow to keep that cloud going. Once the downburst begins, once the cool air begins to flow down and out, the supply of warm air rising is cut off, and this storm dies.
If you are a few miles away from this storm, you might hear the thunder coming from the lightning. And then several minutes later, there may be a cool gust of wind that comes to you from the direction of that thunderstorm. That's this cool air falling out of the bottom of the cloud. You can tell the outflows coming from each of these storms as it progresses through the afternoon or the evening. You'll feel these outflows reaching you.
Severe Thunderstorms [00:36:36]
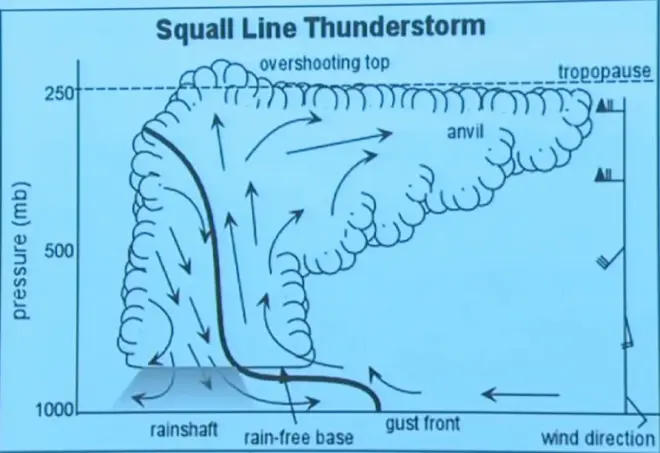
But first I want to start talking about the severe thunderstorms. Now, severe thunderstorms require some of the same ingredients. You need to have a warm, moist atmosphere, no strong inversions, because you've got to get the air moving upwards. But the additional element determining that you will get a severe thunderstorm instead of just an airmass thunderstorm is wind shear in the troposphere. Instead of getting this thunderstorm building up and retaining a kind of symmetry, instead, as it builds up, it gets tipped over by the shear.?
Now, what's different about a severe thunderstorm is that because it's tipped over and made more complicated, the inflow continues on one side of the storm, while the rain and the outflow is on the other side of the storm. They don't cancel each other. Because it's sheared in this way, you get air coming in from the back that gets caught up in the rain, brought down to the surface to form the cool air and the gust front. And then out ahead of the gust front comes the warm air, which is the fuel for the thunderstorm, that'll rise up in this way and spread out to the right and a little bit to the left.
So the downdraft caused by evaporation and the updraft, which is generating the cloud, do not--they're not in the same place, so they don't cancel each other. So this can be a long-lived storm. This can exist for many hours, just trucking across the landscape, sucking up air from one side, spitting it out the back along with rain, and continuing to kind of move along like that until conditions change, until it encounters air that's too dry or whatever might change, might eventually kill the storm. But it could even last overnight. In other words, it could start this afternoon, and the same storm could exist through the night--in some cases, even into the next day--because it doesn't have a way to naturally kill itself off.
Also, these are much more severe. They are broader in extent. The updrafts are quite a bit stronger. Instead of being two or three or four meters per second updraft, they could be 10 or even 15 meters per second updraft speed. And this gives rise to a whole set of new dangers that come along with this storm.?The storm is?going to move by the direction of the winds in the middle of the troposphere. Typically, the winds around 500 millibars will determine how that storm moves. So normally, from west to east, but it'll depend on the local conditions. Could be in some other direction if the regional winds are from a different direction.
What damage do you get from severe thunderstorms? Heavy rain and flooding. The rain rates can be very high. And again, if they’re moving—if the storm's moving slowly, that's the most dangerous situation, because it'll put a lot of water into each watershed.
Because the updraft speeds are so large and there's a lot of supercooled water above the zero degrees Celsius line, you can develop riming, which will then grow and grow and grow, and that hailstone can be suspended for maybe 15 or 20 minutes, even as it grows, because of the very strong updraft speed. So it'll continue to grow until it finally gets too big, and then it'll finally fall out. But by that time, it may be quite a chunk of ice that has grown by riming. And then as that falls to Earth, it picks up a lot of speed, and that could cause damage to crops, buildings, or people.
There will be usually hundreds if not thousands of lightning strikes from these severe thunderstorms, and that's a real danger. And so these are big lightning producers.?It has to do with ice crystals bouncing into each other and then being carried off by different vertical motions. When they strike into each other, they separate charge. And electrical charges of one sign get carried up, developing a big voltage difference between the upper part of the cloud and the lower, or the cloud and the ground. When that breaks down, you'll get lightning strikes.
Tornadoes are formed by severe thunderstorms.?These?large, severe thunderstorms generate a slow rotation within them. That's called the mesocyclone, or the mesovortex. We understand how that forms, but that's a slow, large-scale vortex. It might be 40 or 50 kilometers across and with wind speeds of only 4 or 5 meters per second. That certainly isn't the tornado itself, but it may be that somehow that rotation gets amplified near the surface of the Earth in certain spots that would generate tornadoes.?So it may be the mesovortex is the precursor of the tornado itself, but that's one of the things we've been unable to determine with scientific certainty.?
The recent recommendation from the National Weather Service is that that probably is not valid, or at least it's not--probably, by opening the windows, the chances are you'll do more damage to your house than by keeping them closed. Either the wind will come in or rain will come in and you'll get damage that way. There's no real strong evidence that this pressure difference has an effect on exploding your house.
The gust front winds, so that cool air that's generated by evaporation below cloud base, will fall to the surface of the Earth and then spread out at speeds that can almost approach the speed of the tornadic winds. So the first thing that a meteorologist will do when there's been a severe thunderstorm and damage has been reported, he'll get in his car or he'll get in a helicopter and he'll scout the area and try to figure out was the damage due to the gust front winds or from the tornadic winds??That's question number one whenever these kind of storms occur. Can you separate the damage caused by that cool downburst of air from the damage caused by the tornado itself?
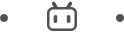