05. How Selection Changes the Genetic Composition of Population
EEB 122. Principles of Evolution, Ecology and Behavior

Lecture?05.?How Selection Changes the Genetic Composition of Population

https://oyc.yale.edu/ecology-and-evolutionary-biology/eeb-122/lecture-5

What we're talking about today is a look into a very basic mechanism that is operating in all of life and is causing the accumulation of information. There are four major genetic systems, and there are some interesting exceptions to them. They are sexual versus aSexual and haploid versus diploid, and those differences make a big difference to how fast evolution occurs. When adaptive genetic change starts to occur, it is virtually always slow at the beginning, fast in the middle and slow at the end.?
Darwin?had a model of blending inheritance. That meant that he thought that when the gametes were formed, gemmules from all over the body, that had been out there soaking up information about the environment, swam down into the gametes, into the gonads, carrying with them information about the environment into the gametes, and that then when the zygote was formed, that the information from the mother and the information from the father blended together like two liquids.?In other words, he didn't think of genes as distinct material particles but rather?fluids.?The problem with blending inheritance is that the parental condition gets too?blended out to?preserve?information.
That's why Darwin came under attack. And Mendel wasn't known, and he resorted to Lamarckianism, and he was wrong. So genetics became an issue. In the year 1900 there was a simultaneous rediscovery of Mendel's Laws, and at that point people went back and they read Mendel's paper, and they realized that they had missed this 35 years earlier.
Then the so-called 'fly group' of Thomas Hunt Morgan and Sturtevant and Bridges, who were working at Cal-Tech, demonstrated that genes are carried on chromosomes. And enough then was known about cytology, so that we knew that chromosomes had an elaborate kind of behavior, at mitosis and meiosis, and people then, about 1915, showed that in fact the behavior of chromosomes was consistent with Mendel's Laws.
However, there were still issues about whether all of this would actually work at the population level. It was not immediately clear that you could take Mendelian genetics and then construct populations out of it, that obeyed Mendel's Laws, and have natural selection work. To do that actually required a fair amount of math, and the people who did it were Ronald Fisher, J.B.S. Haldane and Sewall Wright?between about 1918 and 1932. They?invented basic statistics to?lay?the foundations.?Fisher had to invent analysis of variance in order to understand quantitative genetics, and Wright had to invent path coefficients in order to understand how pedigrees translate into patterns of inheritance.?As a result of that, genetics really became regarded as kind of the core of evolutionary biology during the twentieth century.

The genetic system of a species is really the basic determinant of its rate of change. So we have Sexual versus aSexual species--there are complications to this--and we have haploid versus diploid, and there are other ploidy levels.?
Basically the difference between Sexual haploids and Sexual diploids is the point in the lifecycle where meiosis occurs.?
If the adult is diploid and meiosis occurs in gonads in the adults that produce gametes, and then the zygote form develops so that all of the cells in the developing organism are diploid, you get the diploid cycle.
If you have the zygote having meiosis immediately, or shortly after being formed, so that the developing young are haploid, then you get a haploid adult. So this is what moss do and this is what we do.
Then we have aSexual haploids and aSexual diploids, and at least in outline they look pretty simple. ASexual diploid, just makes a copy of itself; just goes through mitosis, makes babies. ASexual haploid, same kind of thing.
Those are the four major genetic systems. There are many, many variations on them. ASexual types are not frequent among vertebrates. They are common in plants. Most bacterial Sex is aSexual, although bacteria do have a bit of Sex. People are?diploid; your adult large form is diploid. Mosses are haploid.?
The aSexual haploids are things like the tuberculosis pathogen, blue-green algae, the bread mould, the penicillin fungus, cellular slime moulds, and they constitute the bulk of the organisms on the planet.
Sexual haploids are things like moss, and red algae; most fungi are Sexual haploids. In this case you can see that's where the haploid adult is in the lifecycle. There are where the gametes are formed. They are formed up on the head of the adult. You can see the pink and the blue are coding for the male and the female gametes, on different parts of the gametophyte. Then the zygote forms where the sperm gets into an ovule, on the tip of the plant, and then the young actually develop up here. So this is haploid up here and then the spores go out--meiosis has occurred in here and the spores go out as haploid spores. So that is a Sexual haploid lifecycle.
The aSexual diploids include the dinoflagellates; there are about ten groups of the protoctists--that's the modern name for what you think of as protozoa, but it also includes some single-celled organisms that have chloroplasts in them--the unicellular algae, some protozoa, some unicellular fungi. There are a lot of multi-cellular animals that are aSexual diploids, and this one here, the bdelloid rotifer is one of them. It is called a scandalous ancient aSexual.
People are Sexual diploids, this bee is a sexual diploid, and that flower is a Sexual diploid.?So about twenty animal phyla are Sexual diploids. Many plants, most multi-cellular plants are, and there are some algae protozoa and fungi that are Sexual diploids. They include the malaria and sleeping sickness pathogens. There are some things that don't fit; the Sexual diploid part doesn't fit, for malaria and sleeping sickness.
The things that are alternating between being haploid and diploid, with neither one dominating, are mushrooms, microsporidian parasites, which are things that are actually quite common in many insects, and the malaria--malaria has a very complex lifecycle. So it is haploid inside your red blood cells, it's diploid at a certain point in a mosquito, and it's moving back and forth.
The things that alternate Sexual and aSexual reproduction: there are some rotifers, some cnidarians, some water fleas, some annelids. There's a great little annelid that lives in the bottom of the Harbor of Naples in Italy, and it actually does everything. It can be aSexual--the same species--it can be aSexual; it can be born as a female and turn into a male; it can be born as a male and turn into a female; and it can be born as both and do both. So some things are really flexible, but most things aren't. And the timing of Sexuality and aSexuality is an important part of the lifecycle of all of these things.?

Genetics constrains evolution, and genetics is doing something to evolutionary thought which is about what chemistry does to metabolism and structure, and is about what physics does to chemistry.?If you want to understand molecular and cell biology, you learn a lot of chemistry.?
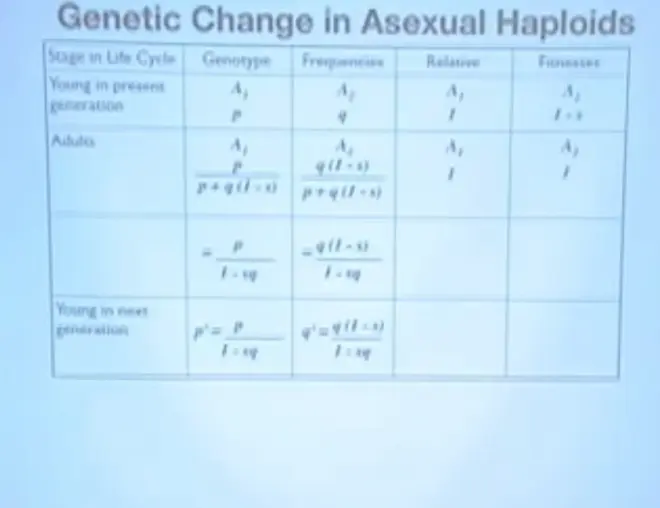
We're going to represent these ideas by symbols. We're going to call alleles Aa. So those are two alleles at one locus; a little exercise of genetic terminology.
We're going to let p be the frequency of A1, and q the frequency of A2. And frequency just means the following: some traits are Mendelian, which means that they're easily recognized in the phenotype. And by the way, the frequency of the other one is going to be 1 minus that frequency, because p plus q is equal to 1; and we'll let s be the selection coefficient, which is measuring the reproductive success of the organism carrying this trait, the difference that it makes.
And if we look at the genetic change in aSexual haploids, basically what one does is make a table of the process; and it is moving from young, in the present generation, through the adult stage, to young in the next generation.
We have genotype frequencies--for genotypes A1 and A2 they're p and q--and we have relative fitnesses up here. We're just looking at the juvenile survival difference between A1 and A2. It changes the frequencies of A1 and A2 in the adults. Basically it changes them by reducing the number of A2s. Some of them have died out; that's 1 minus s. Because these are the frequencies in the adults, the young in the next generation have exactly those frequencies, because there is no selective difference in the adult stage.
We're not looking at all of evolution here,?we're looking at aSexual haploids where selective differences only occur in juveniles. What happens is you get a change in the gene frequencies of the adults that result from that process, and then that exact change is passed on to the next generation.?

In the sexual diploids, you've already been exposed to the Hardy-Weinberg Law, this p2?2pq q2?law.?
We have to assume random mating in a big population. The reason you need the big population is so that those p's and q's are actually accurate measures. In a small population they're noisy, but in a big population they are good stable estimates. And if there's random mating, that means that matings are occurring in proportion to the frequency of each type.
So you get a Punnet diagram like this. You have the probability of one of these alleles occurring; and one parent is going to p, the other allele in that parent q. Same for the other parent. These are the possible zygotes that will result from that. This one has probability p2;?this one has probability q2; and these two together have probability 2pq.
The important thing about the Hardy-Weinberg Law is that it implies that there's no change from one generation to the next. The gene frequencies under Hardy-Weinberg don't change. That means that the information that's been accumulated on what works in the population doesn't change for random reasons. If it's going to change, it's going to change because that big population is going to come under selection.
That means that replication is accurate and fair, at the level of the population, just as it is at the level of the cell. Now, of course, gene drift is going on, but we're not so worried here about gene drift, because gene drift is affecting things that aren't making a difference to selection, and we're building models of selection. What Hardy-Weinberg does is tell you if there isn't any drift, if there isn't any mutation, if there isn't any selection, if there isn't any migration in the population, and if you don't have a high mutation rate, things are going to stay the same.
So it gives us a baseline to see the process of selection occurring, but it also means that random mating in large populations preserves information on what worked in the past. These are also the conditions that remove conflict by guaranteeing fairness. So basically the Hardy-Weinberg situation is one in which everything that was in the population last generation has exactly the same chance of getting into the next generation, in proportion to its frequency; nothing is going to change.?

We go back to John and Jill. They've fallen in love, they want to get married, but they're worried. What's the probability that they will have a child that dies from this disease in childhood?
John's brother died of a genetic disease.?It's recessive?and?lethal, killing?anybody that carries it before they can reproduce.
Jill doesn't have any special history of this disease in her family, but that history's not well known. We estimate the probability that Jill carries the disease from the frequency of deaths in the general population, and that frequency is 1%.


You look at genetic change, in aSexual haploids, Sexual diploids, and it's slow at the beginning, fast in the middle; it's slow at the end.?
The?haploids change faster than the diploids.?Every gene is expressed, and there's no dominance covering up any hidden genetic information. The genes are exposed to selection, in haploids.
The dominants change faster than the recessives. The heterozygotes react like the dominant, but contain the recessive. And so if you're measuring the rate of evolution as the rate at which the dominant takes over the population, it's carrying along in the heterozygotes a bunch of recessives. So development, which is covering up the difference between the two, is actually giving the recessives an advantage and slowing down the rate at which the dominant can take over.
Recessive diploid would be the slowest. If we have an advantageous recessive gene, it gets slowed down by the fact that when it's in the heterozygote, its effects are being covered up by the other allele.
Why is it S-shaped? Why is the trait--let's do it for a dominant diploid Sexual, slow at the beginning, fast in the middle, really slow at the end.?
If you're a dominant diploid Sexual and you're at a frequency of .9, 81% of you are going to be dominant homozygotes; 18% of you are going to be heterozygotes; and 1% of you are going to be recessive. There are eighteen times as many heterozygotes as there are recessive homozygotes. Selection, at that point, is trying to eliminate that 1% of recessive homozygotes. It can't touch the 18%.
If you carry that process over, where we're dealing with .01 and .99, it gets even more extreme. A tinier and tinier fraction of that population is a recessive homozygote. A larger and larger fraction of the remaining recessive alleles are tied up in heterozygotes, where selection can't operate. So this thing just slows way down. It gets harder and harder to get rid of the disadvantageous alleles, because a larger and larger proportion of them--not an absolute number but a larger proportion of them--are hidden in the heterozygotes.
The same thinking describes why evolutionary change in a recessive diploid, where the recessive gene has the advantage, is very slow at the beginning. If a new recessive mutation comes into the population, it's a very low frequency. Its frequency is 1 divided by the number of individuals in the population. The only things it can mate with are dominant forms. All of its babies are heterozygotes.
So at the beginning selection can't operate on it at all. Only after two heterozygotes manage to get together and mate,?will they have a chance to have a?baby that is a recessive homozygote that selection will operate on. So it takes awhile to get this going. And because of dominance, it takes a long time to build up to the point where it accelerates. But then at the end it's fast, because at the end the thing that's being selected is the recessive, and it speeds up as it goes through.
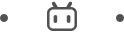