地球發(fā)射入軌彈道設(shè)計基礎(chǔ)(for KSP-RO)
地球發(fā)射入軌彈道設(shè)計基礎(chǔ)
—— Kerbinator?利用 KSP+RSS+RO的研究
(?甚至可以是真實的火箭,盡管有些不太現(xiàn)實 )
來自作者的善意提醒:
*你可能沒有足夠的錢來建造一個(笑),但你仍然可以在模擬中完成它!
**這篇文章不包括火箭制導(dǎo)單元中的實際PID回路控制編程,但它確實給出了正確的上升俯仰程序。您可以編寫kOS腳本(在KSP-RSS-RO中)以提高精度。此外,這篇文章不是非常定量的,它只寫在定性層面,因為它無法使用算法精確計算精確的最佳優(yōu)化軌跡;要為特定的火箭制定正確的俯仰程序,你應(yīng)該按照我的指示,模擬5-10次,直到你的軌跡完全優(yōu)化,也就是說,你的第一級俯仰偏離前進方向的角度不要超過5度,你的第二級可以有一個靜態(tài)俯仰,一直到低軌軌道,其遠點和近點之差不超過50公里,近點高于140(160)公里。
關(guān)鍵詞:地球軌道, 上升軌道, TWR , SLT , delta-v , Isp
????現(xiàn)實的火箭可以將輕或重的有效載荷輸送到不同的軌道,而初軌道,也就是說這些航天器直接進入的第一個軌道通常是近地軌道(LEO)或地球靜止轉(zhuǎn)移軌道(GTO)。然而,有效載荷質(zhì)量對火箭結(jié)構(gòu)和上升彈道有很大影響。這里我們只關(guān)注兩級火箭的上升彈道,我將分兩部分來解釋,理論和例子。第一部分我將解釋理論,第二部分我將提供一些例子來說明它是如何工作的。
????這里的主要理論因素是TWR、delta-v和Isp。首先,TWR(或SLT)顯示了飛船對抗重力的能力。隨著TWR的提高,你將能夠更快地上升并輕松獲得更高的垂直速度。所以這就是為什么第一級通常具有1.2至1.5的高SLT,基本上是因為它們垂直升空,推力必須克服重力,所以TWR必須高于1,但如果你不想在發(fā)射開始時損失大量Δv,隨著你不斷消耗燃料,飛船變得更輕,但由于發(fā)動機結(jié)構(gòu)不可改變,推力絲毫沒有降低,你的TWR將大大增加,通常在MECO達到6-7。我還想指出,峰值G力對宇航員和航天器(尤其是前者)是有影響的,所以即使是土星五號的SLT也很差,只有1.13,但就在MECO之前,他們在MECO前幾秒鐘關(guān)閉了一臺F-1發(fā)動機,但這實際上并不會降低其效率,因為如果TWR超過4,則幾乎沒有差別——重力幾乎可以忽略不計。(太高的)TWR也會影響火箭的效率,因為TWR越高,發(fā)動機的結(jié)構(gòu)質(zhì)量就越高,更高的結(jié)構(gòu)質(zhì)量意味著delta-v的降低,因為你會得到更高的起始質(zhì)量和更高的終止質(zhì)量,所以dv會降低。此外,HLV的一個有趣之處是,它們通常具有非常低的初始TWR,只有大約1.1-1.2。這是因為,首先,推進劑比發(fā)動機便宜得多,因此,這可以被視為一種具有額外燃料的高SLT火箭,有助于它獲得一定的速度和高度。更重要的是,它以相對較低的速度離開稠密的低層大氣,可以降低施加在火箭頭部的動壓,保護火箭不因空氣動力而像挑戰(zhàn)者號航天飛機的軌道器之類的被撕裂。同時,它還可以防止在MECO之前出現(xiàn)極高的重力峰值。但一些MLV,如阿特拉斯551和德爾塔II/Delta IV M,并不遵循這一規(guī)則——它們通常通過使用安裝在側(cè)面的一些小型固體火箭助推器來實現(xiàn)良好的起飛TWR,以幫助第一級從地表以足夠的速度起飛沖上天空。盡管固體火箭助推器的Isp比液體燃料發(fā)動機的Isp低,但由于其重力損失比低TWR火箭低,因此仍然提高了效率。更先進的解決方案是CBC設(shè)計,用于Delta IV重型和Falcon重型火箭,使用三個平行的相同大型液體燃料助推器,中央核心節(jié)流閥降低,使其在兩側(cè)助推器后燃燒。
????第二個因素是Δv。盡管Δv不是凈速度變化,但它與考慮計算重力損失的(凈)速度變化量直接相關(guān),特別是(取決于)MECO速度。MECO速度決定了第二級的最低初始TWR。例如,如果MECO速度僅為2.7 km/s左右,如SpaceX Falcon Heavy,第二級TWR必須至少為0.9,但如果你的MECO速度是4.3km/s,就像大多數(shù)真正的HLV一樣,你就可以將第二級的TWR降低到0.36。這是因為MECO速度越大,第二級繞軌道運行所需的時間就越短(同一推重比下),因此重力損失也就越小。從另一個角度來看,第一級的水平速度越快,第二級獲得的離心力越大,第二級受到的重力拉力就越小。因此,盡管只有1.5km/s的速度差,僅為delta-v總消耗量的18%,但第二級的所需初始TWR卻存在巨大差異。
第三個因素是Isp。這不是上升彈道的一個主要因素,但它以一種特殊的方式影響上升彈道。這是因為,在相同的推力下,如果Isp更高,你將獲得更低的推進劑通量,TWR的增加將更慢。例如,起始TWR為0.3,相同的6000 delta-v,讓我們比較液氫NTR(1200s Isp)級和UDMH-NTO(300s Isp)化學(xué)火箭之間的質(zhì)量變化。參考火箭方程:delta-v=Isp*ln(mi/mf),其中mi和mf代表初始和最終質(zhì)量,如果它們一開始都是500t和0.3TWR,讓我們假設(shè)地球G為10m/s2,因此,NTR級的最終質(zhì)量為303.265t(=500/e^0.5),LH2 LOx級的最終重量為67.67t(=500m/e^2)。因此,最終TWR為0.4946和2.216,平均TWR分別為0.385和0.815,這是一個非常大的差異。因此,如果你使用的是D4H方法(我將在后面提到),當向東發(fā)射時,如果你用的是初始TWR 0.28的LH2-LOx上面級,你的GTO軌道的長軸將與你的發(fā)射點與地球中心成60°角,但如果你使用的是氫NTR上面級,初始TWR也為0.28,角度將變?yōu)?0°。
我已經(jīng)建造了三艘假想的火箭,我將以它們?yōu)槔嶋H上,第一艘是典型的LLV,第二艘是專門設(shè)計的HLV而缺乏現(xiàn)實生活中的對應(yīng)物,第三艘主要是為了特殊需要,將飛船直接注入高能軌道,其設(shè)計比真正的Delta IV重型要激進得多。
第一個是QueQiao LV,這是一種典型的輕型運載火箭。它被設(shè)計為攜帶750公斤左右的有效載荷到TLI。它還有一個單獨的第三級火箭發(fā)動機,用于在到達LEO后一段時間燃燒TLI,因此計及TLI火箭發(fā)動機的質(zhì)量,其LEO有效載荷約為2.1噸,我們只關(guān)注LEO上升部分。
在該火箭的設(shè)計中,由于歷史上完全缺乏200kN級的地面優(yōu)化發(fā)動機,我使用了一臺真空發(fā)動機(俄制RD-0124,1990年代),它在真空中提供了良好的359s Isp,但在海平面上提供了中等的245s Isp(245s海平面Isp比地表優(yōu)化的發(fā)動機差得多:地表優(yōu)化的發(fā)動機大約為302s,但比大多數(shù)真空優(yōu)化發(fā)動機好得多,因為大多數(shù)真空優(yōu)化發(fā)動機在海平面的Isp低于200s,這太差了,無法使用)。所以我選擇了西昌發(fā)射中心,它的高度很高,Isp和SLT都大大增加,比在同一緯度但海拔低很多的卡角發(fā)射效率更高,這要歸功于更高的Isp(更高的delta-v)以及更高的SLT(更低的重力損失)。
事實上,這種火箭的TWR相當高,因為Isp和推力都隨著高度的快速增加而增加,因此助推器和芯一級的最終加速度都非常高,都高達7G。
第二級TWR也很高(0.8是令人驚訝地高,除了SpaceX的Falcon Heavy,因為MECO速度太低,只有3km/s的地表速度)。這是因為所有發(fā)動機都有較大推力,而S1.5400/RD-58系列的Isp非常高,2000年左右的RD-58M-CCN達到了驚人的361s,而普通液氧煤油發(fā)動機,真空機通常具有340-345s的真空Isp,而高級的地面機通常為336-338s。
因此,軌道變成了一條連續(xù)上升的曲線,在軌道插入點的末端變得非常平坦。下面是上升曲線:對于第一階段,以100m/s的速度將速度矢量調(diào)高到高于地平線85度,然后跟隨速度順向prograde向上或向下調(diào)1-2度,使得700m/s時速度矢量相對于地平線向上為45度,助推器分離時為25度,然后在助推器分離時盡量取得700m/s的上升率,當速度矢量下降到地平線10度以下時,向上俯仰一點。這樣,你將精確控制MECO時上升率為460-470m/s。然后繼續(xù)推進,直到地表速度達到6.2km/s時下降率降低到20m/s以下。然后俯仰3度左右,使垂直速度始終精確為零。然后,你將進入141-160km的圓形軌道。
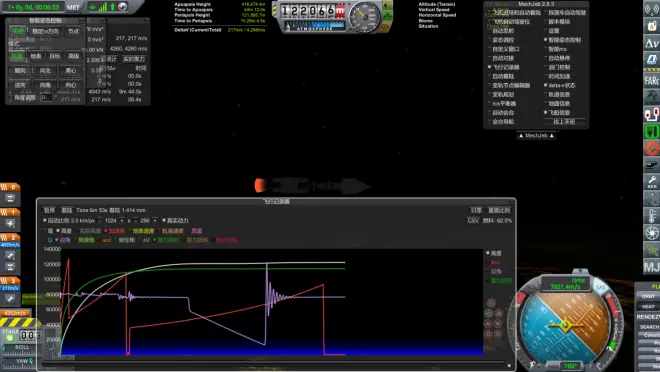
*紫色線表示船的迎角的垂直部分。
下一個,Moon Odyssy HLV,是一種重型先進火箭,用于運載一個無對接的5噸月球著陸器。(*注意,甲烷為燃料的固體核心核推的Isp是不現(xiàn)實的;實際Isp可能在500s左右,這聽起來不是好消息,因為甲烷的效率僅為LH2的1/2.828,但帶有LOx補燃的LH2核推具有類似的推進劑密度和Isp,可以作為替代品。這實際上是模擬中的錯誤)
我使用了一個半假想的RO Raptor(Surface Variant)發(fā)動機(中使用了埃隆·馬斯克的會議的某3500kN的PPT信息),甲烷核推作為第二階段。
由于第二級點火TWR相當?shù)停谝患塪v也不夠(第二級效率遠高于第一級,因為它的Isp高得多,所以增加其(在總dv中的)占比)。因此S2上升相當困難。
另一件惱人的事情是,S1的SLT也很差,只有1.18。這在許多重型運載火箭中很常見,比如土星五號,其SLT極低,1.13,在上升開始時,幾乎88%的dv都被重力抵消,當它從發(fā)射臺緩慢上升時,會造成巨大的重力損失。然而,添加更多的燃料實際上比增加發(fā)動機便宜得多。如此低的TWR主要是出于經(jīng)濟原因。
因此,這里的策略是在開始時輕輕轉(zhuǎn)彎。這枚火箭的上升曲線如下:我以40、70、130m/s的速度一個接一個地轉(zhuǎn)彎3度,這樣它的地表速度在100m/s時恰好指向85度。從那時起,只需保持它在速度順向prograde上仰1度,這樣即使由于地平線上的仰角降低使得200-500m/s時重力轉(zhuǎn)彎速度變得非???,它仍然能夠在地速1000m/s時保持其相對于地平線45度的俯仰,這是一個重要的檢查點。
然后,我將在地速3.2km/s是達到MECO,在300km高度達到170km的遠點。因此,這艘船將有一個非常大的垂直速度,超過1km/s,以幫助低TWR的2級通過消耗垂直速度對抗重力,并獲得足夠的圓軌時間。
然而,僅使用第1級的垂直速度是不夠的。因此,第2級仍需要大量抬頭,以保持高度,防止飛船墜落過快,并因大氣嚴重過熱而導(dǎo)致劇烈爆炸。我從實驗中得出,對于在約310km進入低軌來說,(相對于速度順向prograde)28度抬頭幾乎是完美的,當上升過程中達到的最大高度為350km時,該船實際上在到達Ap后很長一段時間才進入了低軌軌道。這純粹是因為第2階段的低TWR:像這樣的飛行器不可能在到Ap之前以如此小的1級dv和二級推重比直接圓軌。在這里墜落并不重要,飛船仍然遠離大氣層。(然而,這對于載人任務(wù)來說會出現(xiàn)一個”小”問題:如果發(fā)動機因意外而失效,任務(wù)被迫中止,飛船將進入高亞軌道再入,這將產(chǎn)生一個極端的G力峰值:現(xiàn)實歷史的蘇聯(lián)Soyoz 18a由于發(fā)射失敗而中止,因此它在192km Ap的亞軌道進入大氣層,而G的峰值已經(jīng)有驚人的23G,這給宇航員帶來了生理不適;如果Ap是280km,正如我在該飛船的猛禽發(fā)動機回收過程中測試的那樣,峰值重力將超過40G,持續(xù)約10秒,足以殺死宇航員;幸運的是,目前大多數(shù)發(fā)動機都非??煽浚?/p>
圖2 Moon Odyssey HLV的遙測數(shù)據(jù)(aod表示飛船垂直部分的絕對攻角)
注意,隨著時間的推移,aod會有一些變化,因為我是在Smart A.S.S的幫助下手動控制它,而我使用的aod(大約28度)是一個實驗值,并不精確
更激進的方法是D4H-Ex(超過ULA的Delta IV Heavy)上升彈道(僅用于貨運任務(wù);出于與上述相同的原因,我估計任務(wù)中止后的最大減速G-Force將超過100G,將可憐的宇航員壓成薄餅并導(dǎo)致立即死亡),其目的是在不進入低地球軌道的情況下直接注入GTO。一級會大幅度抬頭,同時讓火箭達到極高的遠點和極高的上升率,然后第二級將只較小抬頭(速度順向Prograde向上約10度)并燃燒較長時間,最后直接進入大橢圓軌道,如地球靜止轉(zhuǎn)移軌道(GTO)或地月轉(zhuǎn)移(TLI)。在這種方法中,不可能注入低地球軌道,但可以使用非常低第二級TWR,低至0.28(這個值幾乎是固定的,因為我的實驗得出了這個值,0.29會使Pe過高,0.27會進入大氣層并燒毀,導(dǎo)致災(zāi)難性失敗)。如果您使用NTR,它將非常好,因為NTR非常重且高效,這意味著NTR(Isp 622s)所需的7500 m/s并不太多,但它在化學(xué)階段(Isp 320-460)中這個dv是極高的。因此,它通過在核推級上堆積更多的delta-v來最大化核推級的高效率,所需的低TWR意味著它需要更少的核發(fā)動機,因此它具有更少的自重,因此效率更高。至于歷史上的運載火箭,它們不使用核發(fā)動機,但也使用高能氫氧上面級,與大多數(shù)推進劑的300-330秒Isp相比,它提供了出色的460秒Isp。主要缺點是發(fā)射時間必須精調(diào),因為與離開LEO不同,LEO可以讓你的長軸指向任何你想要的地方,然而,在這個上升彈道中,它是由發(fā)射時間決定的。因此,月球起飛窗口從每天2個限制到每月2個。此外,發(fā)動機必須非??煽浚m合RL-10系列或NTR等長時間燃燒,以便在長得離譜的入軌燃燒過程中不會發(fā)生中途故障。
D4H上升彈道背后的原理相對簡單:當你的速度高于該高度的圓軌道速度(7.6~7.8km/s)時,離心力將超過重力,下降速度將迅速下降。因此,很大的下降率并不重要;事實上,下降率峰值約為-1.2km/s,遠高于俯仰上升以保持高度的方法的下降率,其中當初始TWR為0.45(我的實驗值,使用核推)時,最大下降速度不能超過500m/s,這是俯仰上升方法可用的最低實際TWR。
先前在簡單火箭1已經(jīng)做過初試驗,結(jié)果如下:
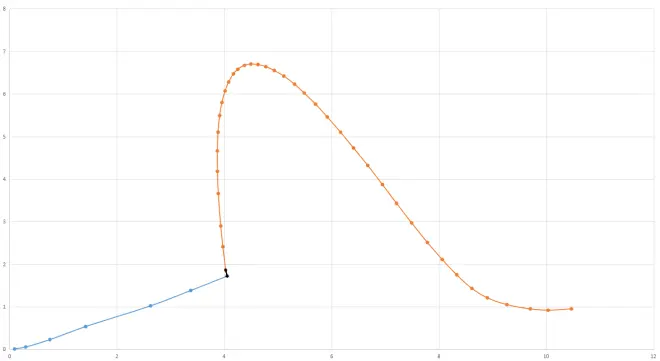
橫軸為速度,單位km/s,縱軸高度,單位百km
因此,我使用了一艘飛船Lunacy(RF,而不是RO,這意味著這些發(fā)動機盡管具有近似現(xiàn)實的性能,但仍然是假想的)。在這個模擬中,彈道方案是,在MECO時保持速度升角為30°左右,并保持相對于速度順向Prograde上仰10°。整個TLI上升的總dv為13.1km/s,遠高于普通火箭的12.2km/s,然而,更少的發(fā)動機在相同質(zhì)量下提供更多dv,更少的引擎意味著更低的總成本,這符合火箭設(shè)計的最終目標。曲線中的最高點是850km。
因此,這些是我的LEO/GTO上升彈道的一些部分,并且由于我使用了一整套擬真mods,所以結(jié)果非??尚?,這可能適用于使用相同發(fā)動機和類似油箱的真實發(fā)射。這些計算機模擬可以節(jié)省大量時間,同時保持極高的準確性。它還提供了一種學(xué)習(xí)火箭技術(shù)的方法,而無需擁有類似SpaceX的公司。因此,在進行粗略估計時,它可以用于指導(dǎo)火箭設(shè)計。
我還想展示,這種方法是可靠的,因為所有結(jié)果在任何現(xiàn)實模擬中都是完全可重復(fù)的(特別是KSP-RO,我在那里開發(fā)了這些假設(shè)火箭:在沒有發(fā)動機故障的情況下,QueQiao LV的成功率超過80%,而Moon Odyssey只有手動控制,成功率超過50%(沒有自動駕駛,但我使用了智能駕駛輔助系統(tǒng),需要手動控制作為數(shù)字輸入)
現(xiàn)在我們已經(jīng)梳理了低地球軌道上升軌道設(shè)計的基礎(chǔ):首先我們需要有9.0-9.6km/s的delta-v,然后我們將根據(jù)火箭的級TWR設(shè)計其上升剖面,然后在模擬中手動飛行,以優(yōu)化您的軌跡,最后,如果第一級能夠正確飛行,將船舶送至所需的高度和速度,而不會因前進而產(chǎn)生太大的俯仰,那么重力轉(zhuǎn)向就可以了,最后,當你將第二級以一定角度俯仰時,它將在160公里以上的高度進入一個近乎完美的環(huán)繞地球的圓形軌道,你的設(shè)計將成功完成。
作者:Kerbinator
用時:121+184+114+62=481min;翻譯為中文+50min
20191101 07:54于Kerbin University of Aeronautics and Astronautics(KUAA)(笑)

關(guān)于實用的彈道設(shè)計標準(二級)
MECO速度 - 二級初始TWR - 抬頭角
2800 - 0.96 - 6°
3600 - 0.5 - 20°
4300 - 0.38 - 45°
0.38是低推重比二級的極限,再低的推重比,抬頭維持高度法將不再適用。
全部為Ap后圓軌,MECO速度越高,二級推重比越低,掉高度越嚴重。
對于核推+普通抬頭圓軌法:
TWR增長太慢,0.38需要修正到0.46,核推二級不建議過低的MECO速度。
墜落圓軌彈道推重比固定0.29-0.33:
墜落圓軌彈道需要高比沖長時間工作的上面級(彈道適應(yīng)性);
更高的推重比會在高處提前Pe出地面,更低的推重比會墜毀。

原始文本展示
原始文本展示如下(其實是大一的英語寫作作業(yè)(笑))
注:無修正。圖片和上面的翻譯展示版相同,已經(jīng)略去。
THIS IS APPENDIX , NOT A PART OF THE ESSAY ! BY READING THIS APPENDIX YOU WILL MEET THE BASIC STANDARD TO READ THIS PASSAGE.
And this passage is written in a textbook/tutorial style.
* Small characters are used to fit the ultra-long passage into a small place huh.
Author:有道翻譯勉強能看(有些地方寫得還挺順的)
A harsh 4h work!--As a part of my planned《introduction to aeronautics and astronautics for KSP-RO players》(←航概 for KSP-RO)
abbreviations:
deg/d:degree(s) (angle)
s1?, s2?... : stage 1?, stage 2?, ?...
LEO:Low Earth Orbit?, 7728.3?m/s at 302.774?km alt?(?typical for HLVs?);?7815?m/s at 160?km or 7824.5?m/s at 140.000km?(?typical for LLVs?)
LLV : light launch vehicle
HLV?:?heavy launch vehicle
TWR : thrust-to-weight ratio
Dv/Delta-v?: the velocity change in total(not for net velocity change?, considering steering losses?, gravity losses?, and change of reference?frame)?. It's the net velocity change when there is completely no gravity and the vessel is keep thrusting prograde?, so in simulation you can cheat to set the gravity to zero in order to?test your delta-v?.
Prograde?/?retrograde:velocity vector's positive&negative direction?.
Apoapsis?:?the farthest point of orbit from center celestial body?(Earth?, shown here?)?. In suborbital trajectories?, the ship ascend before apoapsis?, and descend after apoapsis?.
Statement?:?Well?, despite being a modified video game?, the Kerbal Space Program with Real Solar System?, Realism Overhaul and Principia have a very realistic output?in term of accuracy(provided by Principia’s N-body physics and FAR’s aerodynamic model?) and how things work(provided by Realism Overhaul)?, similar to Orbiter?, another video game ( really !) used by NASA scientists to calculate trajectories?. Also KSP-RSS-RO is capable to do every real space mission so the output is realistic and plausible?.
LEO?:?Low Earth Orbit
GTO?:?Geostationary/Geosynchronous?Transfer Orbit
TLI?:?Trans-Lunar Injection
LH2?:?liquid hydrogen(?a kind of rocket propellant?)
LOx?:?liquid oxygen(?rocket propellant?)
Isp?:?Specific impulse?, which means impulse?(?force?*?time?) the rocket gets with 1 mass unit of fuel(ex. a engine consume 1?kg of LH2?/?LOx propellant and get a 1?t vessel accelerated 4.66?m/s?, then its Isp is 4660?m/s?, and after being?divided by Earth G 9.80665m/s2 in British units?, it became 474s?)
Lunacy?*:?not *?being mad?*?, this is my name on one of my ship as an attempt to a moon return mission carrying a 140-kg kerbal?.
PLEASE read the abbreviation vocabulary first !
Author’s notes:
The first passage?(?Introduction to Rocket Propulsion Technology?)?is merely a collection of existing knowledge?I learned from Wikipedia a year ago?, but this is really my original work based on my own simulation experience , and it is quite simple (?) in comparison with those long essays , and is it far more general , not limited to specific rocket with a fixed ascent profile?suiting its TWR and other properties?.
IF YOU HAVE DIFFICULTY READING?THE PASSAGE , PLEASE TAKE A LOOK?AT THOSE PICTURES , AND TRY TO UNDERSTAND EVERY LINE IN THEM?!!!?IF YOU WANT TO FULLY UNDERSTAND THIS PASSAGE?, PLEASE CONTROL THE ROCKET MANUALLY FOLLOWING MY INSTRUCTIONS?!!!
Fig. A brief explanation of thrust ( right ) and gimbal ( left ) used for rocket control .
Earth Orbit?Ascent Guidance for Realistic Rockets
(地球發(fā)射入軌彈道設(shè)計基礎(chǔ))
—— Kerbinator’s Research with Kerbal Space Program?+?Real Solar System?+?Realism Overhaul?+ Historical Rockets Parts Pack
(?and hopefully?, realistic?rockets despite some inaccessiblities?)
Author’s kind reminders:
* You may not have enough money to build one :D , but you can still do it in simulation!
**?This passage does not include the actual PID loop control programming in rocket guidance unit?, but it indeed gives out a correct ascent pitch program?. You can write kOS scripts (?in KSP-RSS-RO?) to aim for accuracy?. Also this passage is not very quantitative?, it is only written to a qualitative level since it cannot precisely calculate the exact best-optimized trajectory using algorithms ;to figure the right pitch program for a specific rocket?, you should follow my instruction and simulating is for 5-10 times until your trajectory is fully optimized?, that is to say?, your 1st?stage don’t pitch out of prograde for more than 5 degrees?, and your 2nd?stage can have a static pitch all the way to a LEO orbit?whose difference between apoapsis and periapsis is no greater than 50km and periapsis is higher than 140(160)km.
Keywords :?Earth orbit , ascent trajectory , TWR , SLT , delta-v , Isp
????Rockets in reality can deliver a light or heavy payload to various orbits?, and the primary orbit?, which means the first orbit these spacecrafts directly inserts in is usually low earth orbit(LEO) or geostationary transfer orbit(GTO)?. However the payload mass affect rocket structure and ascent profiles a lot?. Here we’ll only focus on the ascent profile of two-stage rockets , and I’ll explain it in two parts , theories and examples . In the first part I’ll explain theories and in the second part I’ll provide some examples which shows how it work?.
????The major?theoretical?factors?here are?TWR , delta-v and Isp?. Firstly , TWR( or SLT ) shows the ship’s ability to fight against gravity . With a higher TWR , you will be able to ascend faster and gain more vertical speed?with ease?. So this is why the first stage usually have a high SLT of 1.2 to 1.5 , basically because they lift off vertically and thrust have to overcome gravity force?so the TWR must be higher than 1?, but if you don’t want to lose a large amount of delta-v?at the beginning of launch?, you will have to get your SLT?at least 1.1?. For instance , if your SLT is 1.2 , that means you will lose 80% of your delta-v due?to gravity pull . However , as you consume fuel constantly the ship gets lighter but thrust does not decrease at all due to unchangeable engine structure , your TWR will increase a lot , and typically hit 6-7 at MECO . I also want to point out high peak G-Force is brutal to astronauts and spacecrafts , so even the Saturn V has a poor SLT of 1.13 , but its TWR just before MECO is so high that to make astronauts comfortable , they turn off one?F-1 engine seconds before MECO , but this does not actually decreases its efficiency , since there is nearly no difference if TWR goes over 4——gravity become roughly a negligible factor . TWR also affects the rockets’?efficiency since the higher your TWR get , the higher the engine’s structural mass will become , and a higher structural mass means a decrease in delta-v since you will get a slightly higher start mass and a much higher terminal mass , so dv decreases . Also?, an interesting thing about HLVs is that they usually have a very low initial TWR , only about 1.1-1.2 . This is because , firstly , propellant is far cheaper than engines , so this can be viewed as a high SLT rocket with extra fuel which help it to gain some speed and a lot of altitude . What’s more?it goes out of dense lower atmosphere with a relatively low speed and it can lower the aerodynamic pressure exerted on the nose of the rocket , protecting rocket from being torn up due to aerodynamic forces like space shuttle challenger‘s orbiter . Also it could prevent a extremely high G-Force peak just before MECO?. But some MLVs like Atlas 551 and Delta II?/?Delta IV M does not follow this rule--they usually have a good liftoff TWR by using some small solid rocket boosters attached on the side to help the stage 1 to kick off from the surface with sufficient speed?, despite the fact that solid rocket boosters’ Isp is lower than that of liquid fuel engines?, it still improved efficiency since its gravity losses is lower than that of low TWR rockets?. A more advanced solution is common core booster design?, which is used on Delta IV heavy and Falcon heavy?, using three parallel identical large liquid fuel boosters?, and the center core throttle down to let it burn up after the two side boosters?.
????The second factor is delta-v . Despite the fact that delta-v is not net velocity change but it is directly related to your velocity change counting gravity loss , especially MECO velocity . MECO velocity determines the second stage’s lowest initial TWR . For example , if your MECO?velocity?is only around 2.7 km/s like SpaceX Falcon Heavy , the second stage TWR must be at least 0.9 , but?if your MECO velocity is 4.3km/s like most real HLVs , you will be able to lower your second stage TWR to 0.36 . That’s because the larger the MECO velocity is , the?less time?the?second stage will take to circularize its orbit , and thus the less gravity losses it will suffer from?. From another aspect , the more horizontal velocity the 1st?stage?pick up , the more centrifugal force the second stage will get , the less gravity pull?the second stage will suffer from . So that’s why despite?having?a?mere?1.5km/s difference which is only 18% of the total delta-v consumption , there is a huge difference in the second stage’s initial TWR .
The third factor is Isp?. This is not a major factor in ascent profile?, but it affect ascent profile in a special way?. That’s because?, with same thrust?, if you have a higher Isp?, you will get a lower propellant flux?, and the increase in TWR will be slower?. For example?, with a start TWR of 0?. 3?, and a same 6000 delta-v , let’s compare the mass change between a liquid hydrogen NTR(1200s Isp)?stage and a UDMH-NTO ( 300s Isp?)?chemical rocket?. Referring to the rocket equation:delta-v=Isp*ln(mi/mf) in which mi&mf?stand for initial and final mass , if they are both 500t and 0.3 TWR at beginning , and let’s make a rough estimation assuming Earth G is 10m/s2 , so the NTR stage’s final mass is 303.265t(=500/e^0.5) , and the LH2-LOx stage’s final mass is 67.67t(=500/e^2) . So the final TWR are 0.4946 and 2.216 , and mean TWR is 0.385 and 0.815 , it’s a very big difference . So if you are using D4H method which I will mention later ,?when launching exactly towards east , if you use a LH2-LOx upper stage with initial TWR 0.28 , your GTO orbit’s long axis will have a 60°angle with your launch point from the center of the Earth , but if you use a hydrogen NTR upper stage also with initial TWR 0.28 , the angle will become 90 degrees .
These theoretical factors mentioned above can be applied to a launch at any planet with atmosphere, like Mars and Venus landers. Now here I provide you some basic facts about an Earth orbital rockets. Firstly the delta-v requirements for a LEO launch is about 9000m/s for high TWR rockets , and about 9500m/s for low TWR rockets . And LEO height is also different, about 160km for high TWR rockets , and at least 300km for low TWR rockets or you won’t have enough time to circularize your orbit . Also , for a reasonable LEO launch , the first stage will need to boost the rocket to at least 3.5km/s to ease the burden on the second stage when fighting with gravity and winning circularization time . To achieve this , you need to have more than 4.5 km/s in the first stage ,and if your first stage SLT is lower than 1.25 , you may need to pack more than 4.8 km/s in the first stage since the gravity loss at beginning will be incredibly high . Make sure to remember all these basic data for a LEO ascent and then we will be able to move on to the analysis of my hypothetical rockets , since I will not mention these basics again , these data will be common sense since then.
I've built three?hypothetical ships?, and I'll take them as example?. Actually?, the?first one?is a typical LLV?, the second one is a specially designed HLV?which lacks real-life counterpart , and the third one is mainly for special needs to directly inject the vessel into a high energy orbit?with a design quite a bit more radical than real Delta IV?Heavy?.
????The first one is QueQiao LV?, a typical light launch vehicle?. It is designed to carry a 750kg-ish payload to TLI?. It also has a seperate 3rd?stage rocket motor to do the TLI burn some time after reaching LEO , so counting the mass of the TLI rocket motor , its LEO payload is about 2.1t and let's only focus on the LEO ascent part .
????In this ship's design?, due to the complete lack of 200kN-level surface-optimized engine?existed in history?, I used a vacuum engine (Russian RD-0124?, 1990s) which offers a good 359s Isp in vacuum but a mediocre?245s Isp?at sea level?(the 245s sea level Isp?is much worse than surface-optimized ones which is approximately 302s but is much better than most vacuum engines which have less than 200s Isp?at sea level which is too poor to use ). So I chose XiChang Launch Center which have a high altitude and increase Isp?and SLT a lot?, making it a lot efficient than launching from Cape Carnival at the same latitude?, but a lot lower?, thanks both to higher Isp(higher delta-v) and higher SLT(much lower gravity losses)?.
In fact the TWR of this rocket is pretty high since the Isp and thrust increase a lot since altitude increase rapidly?, as a result?, the final acceleration of the boosters and center stage 1 is extremely high?, both up to 7gs?.
Also the second stage TWR is very high(0.8 is incredibly high except the Falcon Heavy carried out by SpaceX since the MECO velocity of that is way too low?, only up to 3km/s surface)?. That's because all the engines have a large thrust?, and the Isp of S1.5400/RD-58 series?is really quite high?, up to an astonishing 361s for RD-58M-CCN built around year 2000?, relative to common kerosene-LOx engines?which usually has a?vacuum?Isp?of 340-345s for vacuum-optimized?ones?, and 336-338s for advanced surface-optimized ones?.
So the trajectory turn out to be a continuously ascending curve?which goes quite flat in the end at orbital insertion point . And here comes the ascent profile:For stage 1?, pitch your velocity vector to 82deg above horizon at 1200m/s?, then follow prograde and pitch 1-2 degrees up or down to make the pitch relative to horizon 45deg at 700m/s?, and 25deg at boosters separation?, then focus on ascent rate which is 700m/s at boosters separation?, pitch up a little when velocity vector drop below 10deg to horizon?. This way you will control the MECO ascent rate precisely 460-470m/s?. Then keep thrusting prograde until ascent rate drop below 20m/s at 6?. 2km/s surface?. Then pitch up to about 3 degrees to keep vertical velocity precisely zero at all time?. Then you will get into a 141-160km circular orbit with this for sure?.
Fig.1 Telemetry data record of the ascention of QueQiao LV?. (The orbit is a bit lower due to minor mistakes)
*The purple line indicate the ship’s angle of attack’s vertical part?.
The next one?, Moon Odyssey HLV , is a heavy advanced rocket to deliver a docking-free 5t Luna lander?. (*?Note that the Isp of Methane solid core NTR is unrealistic?;?the real performance may be around 500s?, which sounds not good news?, since Methane has only 1/2.828 efficiency relative to LH2 but a afterburning LH2-LOx solid core NTR?has a similar propellant density and Isp which can serve as a replacement . This is actually a mistake in simulation )
I used a half-hypothetical RO Raptor (?Surface Variant?) engine?( uses one of these information in Elon Musk’s meetings )?, and a methane NTR as the second stage?.
Since the second stage ignition TWR is fairly low?, and the 1st stage dv is not quite enough(2nd stage efficiency is way higher than 1st stage?, since it has a much higher Isp?, so increase its portion)?. So S2 ascent is pretty hard to execute?.
And another annoying thing is that the SLT of S1 is also quite poor?, only 1.18-it's common in many HLVs such as Saturn V?, with a extremely low 1.13 SLT?, which sacrifice almost 88% of dv to gravity at the start of ascent?, causing a great gravity loss when it is rising up slowly from the launch pad?. However adding more fuel is actually?, a lot cheaper than adding engines?. So low TWR is mainly for economic reasons?.
So the strategy here is to turn gently at start?. The ascent profile for this rocket is as follows:I?turn 3 degrees one by one at 40?, 70?, 130m/s?, so that its surface velocity's prograde vector will pitch to 85 degrees at 100m/s?. From then on?, just keep it pitch 1 degree over prograde so that even when the turn became pretty quick at 200-500m/s due to lowered pitch over horizon?, it will still be able to maintain its pitch 45 degrees relative to horizon at 1000m/s surface?, which serves as an important checkpoint?.
Then I'll have my MECO at 3?. 2km/s surface and have an apoapsis at 300km at 170km altitude?. Thus the ship will have a very large vertical velocity?, over 1 km/s?, ?to help the low TWR stage 2 fight against gravity?through?comsuming vertical velocity and gain enough circularization time?.
However?, only using the vertical velocity of stage 1 is not enough?. So the stage 2 still need to pitch up a lot to maintain altitude and prevent the ship from falling too fast and result in a fiery explosion due to severe overheat in atmosphere?. Well I gained from experiments that 28 deg pitch over prograde is nearly perfect for a LEO insertion at about 310km?, when the maximum reached altitude during ascent is 350km—The ship actually insert into LEO well?after the time it reached its apoapsis?. This is purely because the low TWR of stage 2:It will be impossible for a craft like this to enter LEO before apoapsis with such a small stage 1 dv and?. Falling?, at here is not important since you are?, still well out of the atmosphere?. (However it will be a minor issue for manned mission:if engines were disabled by accident and mission was forced to abort?, the ship will go a sub-orbital reentry which have a extreme peak G-Force:the Soviet Soyoz 18a in real life which was aborted due to launch failure?so it?entered atmosphere at an apoapsis of 192km?, and the peak G is an astonishing 23G?which caused minor problems for astronauts;if apoapsis is 280km?, as I tested?during the recovery of the Raptor engine?, the peak G-Force will be at over 40g for about 10 seconds?, large enough to kill astronaut;fortunately most engines are very reliable at present)
(the data recorder exceeded its maximum recording time and I have to break the record?into 2 parts)
Fig.2 Telemetry data of Moon Odyssey HLV( aod indicate the ship’s?absolute?angle of attack’s vertical part?. )
Note aod have a few changes over time since I’m manually controlling it with the assistance of Smart A.S.S.?and the aod I used(about 28deg) is an experimental value , not exact
????A more radical approach is the D4H(Delta IV Heavy by ULA) ascent profile(for cargo mission only;for the same reason as mentioned above?, I estimate that the peak deceleration G-Force after mission abort will be over 100gs?, crushing poor astronaut(s) into pancake(s)?and cause instant death)?which aim to direct GTO injection without entering LEO . This method pitch up stage 1 severely?, and at the meantime get the rocket a extremely high apoapsis and a very high rate of climb?, and then the stage 2 will pitch less(about ~10deg?over prograde) and burn a long time and at last directly insert into a highly elliptical orbit like Geostationary Transfer Orbit(GTO) or trans-lunar injection(TLI)?. In this method it is impossible to inject into LEO but is can use a much smaller TWR of 2nd stage?, as low as 0.28(it's almost fixed as this value come out of my experiment and 0.29 will get periapsis too high and 0.27 will crash into atmosphere and burn up resulting in catastrophic failure?). If you use an NTR?, it will be very good?, since NTR is very heavy and efficient?, which means that 7500 m/s required in NTR (?Isp?622s?) is not too much?, but it is a large amount in a chemical stage(Isp 320-460s)?. So it maximizes the high efficiency of the nuclear stage by packing more delta-v on nuclear stage?and the low TWR needed means it needs less nuclear engines so it has less dead weight , hence more efficient. As for historical launch vehicles , they don’t use nuclear engines , but they also use energetic LH2-LOx upper stage , which offers a excellent 460s Isp?compared to 300-330s Isp?foe most propellants . The major?shortcoming is that launch time must be fine tuned since unlike departure from LEO which can let your long axis point everywhere you want?, however in this ascent profile it is decided by launch?time. So?, moon departure window?, is restricted from 2 per day to 2 per month?. Also the engine must be very reliable ,suitable for long burns like RL-10 series or NTRs , so that they will not break down halfway during?the ridiculously long orbit insertion burn .
????Well the principle behind D4H ascent profile is relatively simple:when your speed is higher than the circular orbital speed at that height?, centrifugal force will exceed gravity and the descending rate will decrease quickly?. Thus high negative vertical velocity is not important;in fact the peak negative vertical velocity is about -1.2km/s?, a lot higher than the method of pitching up to maintain altitude?, in?which the maximum descent rate cannot exceed 500m/s when initial TWR is 0.45?(?my experiment value?)?, the lowest practical TWR for pitch-up method?.
????So?I used a ship Lunacy(?RF?, not RO meaning these engines are still hypothetical despite having realistic-alike performance?)?. In this simulation the profile is to let the prograde maintain a 30d pitch?relative to horizon?at MECO?, and keep thrusting 10d pitched up relative to prograde?. The total dv for the entire TLI ascent is 13.1km/s?, a lot higher than 12.2km/s for common rockets?, however less engines increase dv at the same mass?, and less engine means lower total cost?, which matches the ultimate aim of rocket design?. The highest point in the curve is 850km .
????So these are some parts of my LEO/GTO ascent profiles?, and as I used a full kit of realistic mods?, so the results is quite plausible?, which may be applied to real-life launches with the same engines?and similar fuel tanks?. These computer simulations?, can save?much time while maintaining great accuracy?. It also provide a way to learn rocketry without owning s SpaceX-alike company. So it can be used to instruct rocket design at the beginning when doing rough estimations .
I also want to imply?that?this method is reliable since?all results are completely repeatable in any realistic simulation(especially KSP-RO where I develop these hypothetical rockets:without?engine failures?, the QueQiao LV has a success rate of over 80% and the Moon Odyssey?has a success rate of over 50% with only manual control(no autopilot?, but I used?a smart pilot assist which requires manual control?as numeric input))
Now we’ve sort out the basics of a LEO ascent trajectory design:at first we need to have 9.0-9.6 km/s of delta-v,then we will design its ascent profiles , based on the rocket’s stage TWR , delta-v distribution and Isp of its engines . Then fly it manually in simulation to optimize your trajectory and finally whtn your first stage can fly properly sending the vessel to a desired altitude and speed without much pitching from prograde then the gravity turn will be OK , and finally when you pitch your second stage at a certain angle and it enters a nearly perfect circular orbit around Earth at an altitude over 160km and your design will be successfully finished.
By:Kerbinator(My online name)
At Kerbin University of Aeronautics and Astronautics(KUAA)(Just for fun xD)
TFinal:20191101 7:54 a.m.
BASE:121 min on mobile
Overhaul:184 min on PC
Overhaul 2:114?min
Overhaul 3:62 min

后記
祝賀SLS成功發(fā)射。
本文作為教程,獻給KSP+RSSRO的玩家們,以供作為參考使用。