09. Water in the Atmosphere I
GG 140: The Atmosphere, the Ocean, and Environmental Change

Lecture 09.?Water in the Atmosphere I

https://oyc.yale.edu/geology-and-geophysics/gg-140/lecture-9

Mixing in the Atmosphere (recap) [00:00:00]
We were talking about mixing in the atmosphere. And we did two types of analysis. First of all, we assumed that there was a turbulence in the atmosphere that would mix gases or particles into a larger and larger volume. And we did three problems like that.?
One was adding a material to a confined valley.
The second was adding material to the atmosphere in an unconfined situation. Where the turbulence would act to spread that material out progressively with time.?So the longer the time had elapsed, the larger would be the volume into which the material had mixed. And the more dilute it would have become.
The third problem we did was adding material at a steady rate into an atmosphere that has both turbulence and wind. In that case, the added material forms a kind of a plume downwind of the source point, spreading out as it goes horizontally and vertically, and becoming more dilute for that reason. It spreads out into more and more volume as it moves down wind.
And then I went on to start to talk about the role of the temperature gradient in mixing.?It turns out that the temperature profile in the earth's atmosphere plays a very important role in how material mixes into the atmosphere.?And that arises because under certain circumstances, a parcel of polluted air will be able to rise and spread into a large volume aloft. In other situations, that parcel if it tries to rise, may fall right back down to where it started and be unable to mix vertically. And this has to do with the vertical temperature gradient.
Atmospheric Stability [00:02:36]
I will?define?atmospheric stability as the resistance to vertical motion. And this is a plot of temperature on the x-axis versus height on the y-axis.?And we put on there a reference line, which?is the?DALR. That's the dry adiabatic lapse rate. I call it just the adiabatic lapse rate. But it's good to call it the dry adiabatic lapse rate because inside a cloud, that takes on a different slope. So this is one when you're outside a cloud, there's no water vapor condensing. So we'll call it the dry adiabatic lapse rate.
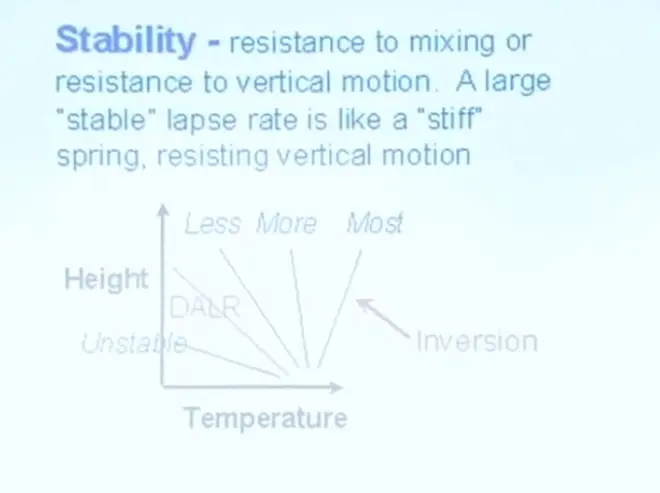
In each case, we're going to want to compare that with this reference curve, the dry adiabatic lapse rate.?All of these that are less horizontal, that is more vertical or more to the right, than the dry adiabatic lapse rate, are going to be called stable. Because if you lift a parcel in the air, it's going to be cooling at a rate of 9.8 degrees per kilometer. That's going to be in all cases colder than the environment into which it's been lifted. If it's colder, it's going to want to sink back down to where it came from. So we call that stable.
The unstable case is where you have a very strong decrease in temperature with height. Then if you lift a parcel along the dry adiabatic lapse rate, it's actually getting warmer than it's environment. So when it's been lifted a little bit, it finds itself warmer than its local environment. That means it's buoyant and will?rise further.
The Diurnal Cycle of the Adiabatic Lapse Rate [00:06:29]
I want to walk through a typical diurnal cycle, a day night cycle.?
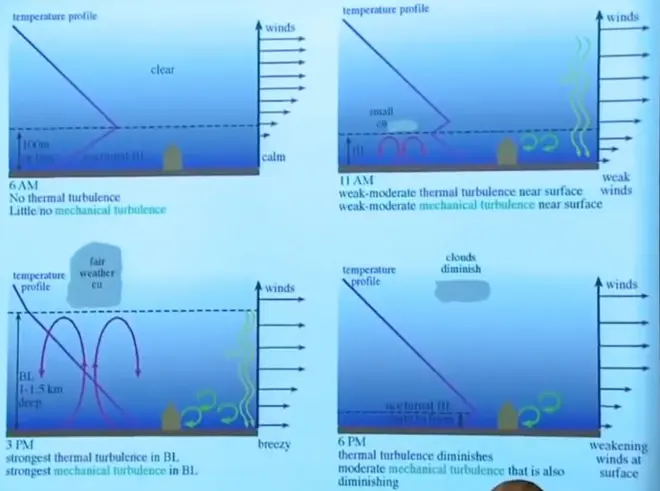
At 6:00 AM this morning, you have built up a nocturnal boundary layer. This is temperature versus height plotted here with the purple curve. Notice that there's a positive lapse rate in the lower part of the atmosphere in the early morning.?The ground has been cooling off all during the night by radiating infrared radiation to space. That's drawn heat out of the lowest few meters of the atmosphere. And has produced that positive lapse rate. It?is a surface level inversion. It's an inversion located right at just above the earth's surface. And that typically happens overnight on a clear night when the radiation can leave, can escape the earth's atmosphere without hitting clouds. That's a very stable atmosphere and turbulence will be suppressed. There will be very little turbulence?or?vertical motion. And the air will just lie there quietly in layers.
The wind, which has continued to blow aloft all during the day and night, will be calm at the surface. Because without the turbulence, there's no way to mix that momentum down to the surface of the earth. Remember the wind aloft doesn't feel the diurnal cycle very much. So it blows day and night typically. That depends more on the local weather systems and so on. But the winds often go calm at night at the very surface because of this lack of turbulence to mix the momentum down.
For example, sitting at home 7:00 in the morning,?I?looked out, none of the leaves were moving. And then around 8:00 or 8:30, suddenly the leaves begin to move finally. Because the earth had heated up the atmosphere, convection began, and you start to get the wind being brought down. And the wind starts to move at the earth's surface.
So let's go to that next stage then. So 11:00 AM, the sun is heating the earth. If the earth is getting warm then it's putting heat into the lowest few meters of the atmosphere. And that's going to bring that lapse right around this way, hotter below and cooler above.?If it goes unstable, convection is going to begin. The heat being provided by the surface of the earth produces a strong negative lapse rate, pushes the atmosphere into an unstable state, and convection begins. The air begins to turn over. When it does that, it brings some of that general wind down to the earth's surface.
That trend continues. By 3:00 in the afternoon, that convection layer has grown to a kilometer or a kilometer and 1/2 deep. Very often there are cumulus clouds with their flat bases right at the bottom of that--or right at the top of that convective layer.?So this afternoon, if you look out and you see cumulus clouds, you'll know that this has happened. And that cloud base is right at the top of the convection boundary layer.
And then when we get to the evening again, the sunlight stops. The earth's surface cools with infrared radiation. And you start to build up that surface level inversion once again. So the cycle just repeats day and night, day and night.
Remember the sun's radiation is coming through. The lower part of the atmosphere is generally transparent. And so the sun's radiation is coming through and hitting the earth's surface, warming it up during the day. And that heat is then transferred immediately from the earth's surface to the first meter or so of the atmosphere. And that's what causes that lapse rate to tip over. Because you're adding heat from the bottom.
Elevated Inversion and Pollution [00:13:48]
Now you could also get what's called an elevated inversion. And that's what's pictured here.?
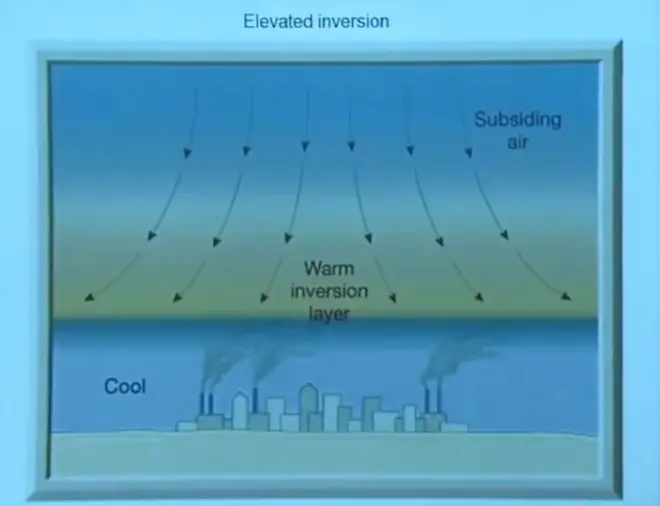
Whenever you have?slow subsiding air up through the troposphere, as you would, for example, as you do today, whenever you have?high pressure and clear skies, you can be pretty certain that there is very?slow subsidence. The air is slowly descending through the troposphere.?That air cannot descend through the earth's surface because the earth's surface is solid. So it has to spread out. You end up in this subsidence situation creating an elevated inversion.
If I plotted the temperature, it would probably look like decreasing, then increasing, and decreasing again in an elevated inversion.?An inversion of this type too is very stable. It's almost impossible to mix air across it. So any pollution put in beneath will be trapped, almost as if it were a rigid lid. But it's not. It's air above and air below. But it's warm air above. And therefore parcels that try to rise up through here find themselves too cold, too dense. And they sink back down. And they can't mix that pollutant up into the free atmosphere.
Most of the pollution episodes are connected with these elevated inversions.?There is an elevated inversion trapping that pollutant, preventing it from being diluted up into the rest of the free atmosphere.?So inversions go together with air pollution episodes. They are very closely linked. If the inversion was not there, this pollution could just quickly mix up into the free atmosphere. It would be much more completely diluted. And you wouldn't have the concentration of sulfur dioxide, and NO, and ozone, and so on that you have in these polluted episodes.
I want to give you three examples of rather famous gas releases that kind of illustrate some of the physical principles I'm discussing today. The first two are going to be brief emissions of toxic gases that just happen to take place at night. If the same thing had happened during the day, it would not have been so much of a problem. But at night remember, you've got this stable, nocturnal surface level inversion that prevents vertical mixing.?And therefore, if you emit something at night, it's not going to be diluted. It's going to stay in high concentrations close to the earth's surface. So unfortunately, these first two cases, Lake Nyos and Bhopal, were both night time releases.
The CO2?stayed in a concentrated form and just spread laterally away from the lake killing thousands of cattle but also hundreds of people as well as they slept. This layer of CO2?just slid over them and then basically suffocated them. Because you can't breathe carbon dioxide.
So rather than getting mixed and convectively diluted, it spread out as a layer to the surrounding houses and hundreds of people were killed as they were overtaken by this layer of methyl isocyanate. And that was a big deal at the time, front page?Time?magazine and so on.
So Chernobyl, a different kind of situation; it was the meltdown of a nuclear power plant. It lasted for several days. So it's not a question of day versus night. In this case, the warm radioactive gases released from that plant did lift upwards into the atmosphere and then the wind carried it to great distances.?So maybe the drawback, perhaps the drawback of having a daytime release is it gets diluted. But it also gets up in the atmosphere where the winds can carry it to a greater distance. And if the substance is diluted to a safe level by that mixing process that's OK.?But in this case, there was so much radioactive material that even though it was spread over large parts of eastern Europe and northern Europe, it still caused damaging levels of radioactivity spread by the winds.?This was mostly radioactive iodine that then settles on the grasses and people get it into their system. And they end up with radioactive induced diseases.
The point here is just to talk about what happens when you put substances into the atmosphere. How does it get mixed around? What conditions control that??Forest fires are a good example. You're burning biomass. You're producing smoke. And depending on the atmospheric conditions, that may hug the surface and move downwind like it's shown there. Or it might rise to a higher altitude and then move across through the atmosphere without polluting the very lowest levels.?Eventually it'll fall back down to the surface. But if it's hot enough material, it will rise and get carried away some kilometers above the earth's surface. Those are the two possibilities with forest fires.
Smokestacks are the same way. Now a smokestack is designed to get this smoke released at a point that's above any surface level inversion. So then it'll be carried quickly into the free atmosphere and become rapidly diluted.?So that protects the local environment from the negative effects of the smoke and the air pollution. But it does?mean that now the material will be carried to greater distances because you've put it up into a level where the winds are stronger. And it will be carried off downwind. As in our little problem we did last time with a point source and the wind carrying it away.
Here's an example. You can't make out the mountain very well. But there's a volcanic plume coming out of it. It's rising to a certain level and then spreading out downwind. And I'd like you to think for a minute about exactly why the plume looks like that??So I pulled off a balloon sounding for Iceland. It wasn't at the moment of that eruption. It was just from a couple days ago. But I think the story is the same.
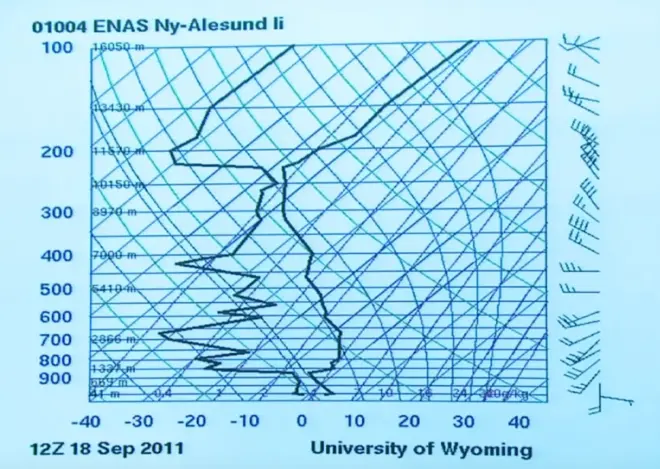
Here's temperature on this axis versus height on that axis. And the temperature is the black curve here. And the?green curves are the dry adiabatic lapse rate.?So if I put in an air parcel at 40 degrees Celsius. The air temperature at the ground is only on that day about plus four Celsius. That air parcel is going to be hotter and therefore buoyant. It's going to rise. As it rises, it's going to cool adiabatically. And eventually, it will come to the same temperature as the environment. At that point, it will have the same density and temperature as the environment. It'll stop rising. And it'll go flat and just move downwind. The point is that a hot, buoyant plume of air will rise, cooling as it rises, until its temperature matches that of the environment. And then it has found it's appropriate level, there's no reason for it to rise further. It'll then spread downwind.
If there was a wind down here of course that would tilt it as it rose. Let's say the wind is from left to right in this diagram. That would tilt this plume. It already has a bit of a tilt. But still it would rise to this level and then move off horizontally.?It'll always follow the wind. But it will continue to rise until it has neutral buoyancy. And then it'll stay at that level.?
Sandstorms can do a similar thing. The winds can pick up--this is off the west coast of Africa. The wind has picked up some dust off the Sahara desert and carried it out over the ocean. And turbulence is keeping it aloft. Eventually, gravitational settling will make that stuff fall back on to the ocean's surface. So you see the same factors at play in this natural phenomenon of just picking up dust from the earth's surface.
Moisture in the Atmosphere [00:29:10]
I want to switch gears and begin to talk about moisture in the atmosphere. These are six alternative ways of describing how much water vapor you have in the air. So in fact, if I know any one of these, I can compute, or in some other way determine, the other five.
One measure of how much water vapor you have in the atmosphere is the partial pressure.?What contribution are the water vapor molecules making to the total pressure of the air? It's probably only a very tiny fraction. If the pressure in this room is at 1,013 millibars, maybe only five or six of those millibars are due to the water vapor molecules.?
The saturation vapor pressure is not actually a measure of how much you have. It's the maximum amount you can have. When you get that amount of water vapor and try to add more, the excess will condense out. And this is a strong function of temperature. I'll be talking about that in just a moment.
The dew point is a measure of how much water vapor you have. If you cool the air down to the dew point then water vapor will begin to condense. So it's the temperature at which water vapor begins to condense out.
The specific humidity is the ratio of the mass of water vapor to the mass of air. It's like a mixing ratio, grams per kilogram, or kilograms per kilogram. For every kilogram of air, how much water has been mixed into it??
The relative humidity is the ratio of the partial pressure of water vapor to the saturation value. It's the ratio of this to this. It's the relative humidity.?That's important because that tells you how close you are to saturation. If you're at 50% relative humidity, that means you could add twice as much water vapor and just then be bringing it to the saturation state.?If you're at a 100% relative humidity, there's already just as much water vapor as can be held. If you added anymore, you would have to condense out.
And you'll recall the wetbulb depression from the lab exercise you did. One way of measuring how much water vapor you have is to have a wet bulb and a dry bulb thermometer. If they read the same, you know there's no evaporation from the wet bulb, which means the humidity is 100%.?If they read differently, that means the air is somewhat dry is able to evaporate water from the wetted wick. And that'll give you a different temperature for the two thermometers. That's the wet bulb depression. And from that you can compute any of these others, except for that one. You can compute those other four.
Condensation is when you change water vapor to liquid. Or sometimes we use that word also when we're condensing it to ice. But usually it's used when we condense it to liquid.?Evaporation is the reverse of that. It's when you take water in the liquid form and evaporate it, put it back in the vapor state.?When you do either of those things, there is heat either taken in or released. And that heat is called the latent heat of condensation. When you're evaporating water, you have to put heat in. When you're condensing water, heat comes out.
I want to make a clear distinction between cloud droplets and raindrops. A cloud droplet is a typical water drop that you see that makes up a cloud. You look up at a cloud in the sky, it is composed of millions of cloud droplets. We put the little suffix “l(fā)et” there to remind us that these are small. A typical cloud droplet is about ten microns in diameter. Ten microns in diameter. A micron?is a millionth of a meter.?A raindrop is a kind of liquid drop you find falling to earth. And they are typically about a millimeter in size. In other words, about a hundred times larger. There is a factor of about one hundred in the size of these two different droplets.
Supercooled liquid water is liquid water that has been cooled down below the normal freezing point. Freezing point for fresh water is zero degrees Celsius.?And yet in the atmosphere, we often find liquid water at temperatures of minus ten, minus 20, even minus 30 Celsius. It's in the liquid state, and yet it is below the normal freezing point of water. We call water in that state supercooled liquid water.?It wants to freeze. It's at a temperature where it should be frozen. But it needs some kind of a trigger to get the freezing process started. And it turns out that plays a big role in the generation of precipitation.
And then this word riming, which is what happens when a super cooled droplet hits something. It wants to freeze. When it hits an object, it'll probably freeze upon impact and stick to the object as a frozen piece of water--piece of ice stuck to the surface. That is called riming, that process of having super cooled water freeze upon impact.
Air Saturation Processes [00:37:17]
The earth, of course, is the water planet. It's covered by large oceans of water. The water gets up into the atmosphere and then in areas where the air is rising, there is adiabatic cooling, which will drop the air temperature, drop the saturation vapor pressure, and bring that air--bring the water vapor in that air to saturation. And as that rising air continues, then the excess water vapor will condense to form cloud droplets.
So you see clouds scattered around in the earth's atmosphere, you can be almost certain that every place you see a cloud, whether it's large or small, there is rising air.?And almost every place you find the sky free of clouds, there is sinking air. Because remember, rising air forms clouds by adiabatic cooling.
So you look at something like this, and well, about half of this is cloud. And half of it is clear. Well, that means half the air is rising. Half the air is sinking. The patterns are complicated. They're connected with a variety of things like fronts, thunderstorms, deep convection over the equator. But in all cases, it's rising motion versus sinking motion--very clearly displayed when you look at a map like this.
So the key thing to understand about clouds then, or about water vapor I should say, is how do we take air that is under saturated or sub-saturated and bring it to the saturated condition??And I'm going to run through the three ways that are active in the earth's atmosphere for bringing air to the saturated state. Remember you're starting with air that has a relative humidity less than a 100%. And you're asking how can I bring it to 100% and above??
Probably the most obvious way is to add moisture, add water vapor to that air parcel.?I'll show some examples of that but sea smoke, contrails, stacks. Smokestacks sometimes give off clouds in this way. And human breath, when you breathe out on a cold day, you can create a little cloud using this mechanism of adding moisture.
Another ways is to cool by removing heat. If you have air that a sub-saturated, but you remove heat, you're going to drop the temperature. If you drop the temperature, you're going to drop the saturation vapor pressure. And you keep doing that, you're going to bring the two together. You're going to bring the amount of partial pressure water vapor together with the saturation partial pressure. They'll be equal. And you'll have the saturated condition.
The third method is by adiabatic expansion, that is by lifting air parcels up in the atmosphere.
So first, I'll be adding moisture. This is something called sea smoke. Here's an aircraft carrier looking out over the ocean's surface. It has a very peculiar appearance. It appears to be tufts of cloud torn apart by turbulence but generally hugging the ocean's surface.?What's happening there is you have cold air moving across the ocean's surface. And the ocean is warm. So the ocean is evaporating its moisture into the atmosphere. But then as soon as it does that, the air being so cold with that extra water vapor being added, you've brought the air to saturation. And you start to form these little tufts of cloud or fog.
Here's another example from the Northeastern Labrador current up off the coast of Nova Scotia.?Whenever you get cold air moving over warm water, you are likely to get this situation. Water evaporates from the warm water, adds moisture to the cold air, you've brought that air to saturation. And little clouds appear to form on the surface.
A contrail is that way. A contrail is a contraction for condensation trail.?And here's an aircraft producing four contrails, one from each engine. So you're burning hydrocarbons in the aircraft engine. The chemical byproducts of this burning are carbon dioxide and water vapor. So the exhaust from the engine is part water vapor. You're adding it to this cold air, which was sub-saturated to begin with. But you've added enough water vapor. So now you've saturated the air and beyond. And the excess goes into these tiny condensed particles either liquid or ice. Those are probably liquid as a result of adding water vapor to cold air.
Now later on, those contrails may seem to lose their contrail appearance. They may spread out. They may diffuse. They may go unstable. They may even lead to what looks like natural cirrus clouds. But even that more natural looking cloud, I think, on this particular day started out as a contrail. It just spread a bit by turbulent diffusion.
If you have a cooling tower like from a nuclear power plant, that's not smoke that's coming out of it. That's just hot, moist air. You're adding water vapor to a dry atmosphere bringing it to saturation and causing what appears to be a cloud. Again that's not smoke. That's just condensed water in the cloud.
And you, yourself on a chilly October or November morning, if you breathe out, the water vapor that's been added to the air in your lungs suddenly is added to the atmosphere bringing it to saturation. And the excess condenses to form the cloud.
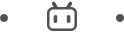