The CRISPR system, its structural features and mechanism of acti
The CRISPR system is a natural immune system of prokaryotes. It is used to fight against foreign DNA, plasmids and phages that invade bacteria. Simply put, viruses can integrate their genes into bacteria and use the cellular tools of bacteria to serve their gene replication. In order to remove the foreign genes of the virus, bacteria have evolved the CRISPR-Cas9 system. Using this system, bacteria can quietly cut off the viral genes from their own genome.?This is a unique immune system of bacteria, which is an acquired immune system of archaea and bacteria to resist the invasion of foreign genetic material.
(1) CRISPR/Cas: A powerful genome editing tool.?
Precise and convenient editing of genes or genomes is crucial for biologists to understand the metabolic processes, gene functions and modern gene therapy in living organisms. Previous genome editing techniques, such as zinc finger proteins (ZFNs) and transcription activator-like effectors (TALENs), have made indelible contributions to the development of genome editing. In these two techniques, the genome targeting of the editing tool relies on the interaction between protein and DNA, while the genome cleavage process relies on the Fokl protein. In Figure 1, the colored circles or ellipses represent the synthetic anchoring proteins (Fig.1). However, a significant drawback of the anchoring process relying on the interaction between DNA and protein is that when a new gene position is to be anchored, the anchoring protein needs to be redesigned and synthesized, which greatly increases the workload and difficulty of gene editing, making these techniques less adaptable to high-throughput genome editing projects.

The emergence of CRISPR/Cas system as an innovative and powerful genome editing tool has changed this situation and can be said to have revolutionized the field of genome editing. CRISPR stands for clustered regularly interspaced short palindromic repeats, and Cas refers to CRISPR-associated. The difference between this tool and the above-mentioned tools is that the CRISPR/Cas system uses RNA-DNA interaction to target genes on the genome, and only a small new RNA sequence is needed to anchor a new gene position. This feature greatly reduces the workload of synthesizing new proteins and has high specificity. The specific mechanism will be introduced in the following text. The reason why CRISPR/Cas system has become the new favorite of the genome editing field is because this tool has many advantages that other tools do not have, such as easy synthesis, convenient use, low cost, high specificity, etc.
(2) The structure of CRISPR/Cas system.?
CRISPR/Cas system is an acquired immune system in bacteria, which is used to fight against foreign DNA, plasmids and phages invading bacteria. Unlike the general immune system of ordinary prokaryotes, CRISPR/Cas system is an acquired immune system, which means that this immune system has “memory”. It can remember the foreign DNA and phages that have invaded before, and cut off their genomes when they invade again. The cut-off genomes will become linear, unable to replicate and express, and be degraded by enzymes in the bacteria. This immune system is simple, but very practical and powerful. What is the structure of CRISPR system? In short, CRISPR/Cas system consists of two major parts: The first part is the genes encoding Cas-related proteins (the white square arrows in Fig.2), which play an important role in acquiring foreign gene fragments and cutting off foreign genes. The second part is called CRISPR array, which contains repeat sequences and spacer sequences, which are interspersed with each other, with two repeat sequences sandwiching a spacer sequence, as shown in Fig.2, where the black diamonds represent repeat sequences, and the different colored squares represent different spacer sequences. The nucleotide composition and length of repeat sequences are relatively conserved and basically unchanged in the same bacteria, but there are some differences between different bacteria. Spacer sequences are used to anchor the target foreign genes, so the nucleotide composition of spacers varies greatly, because they come from different foreign genes. Spacer genes contain highly specific conserved sequences from the anchored genome, ensuring that the RNA transcribed later can accurately pair with the anchored genome. There is usually an A-T rich leader sequence before the CRISPR array, which contains a promoter that is used to initiate the transcription of repeat and spacer sequences. CRISPR array does not contain reading frames (ORF, open reading frame).
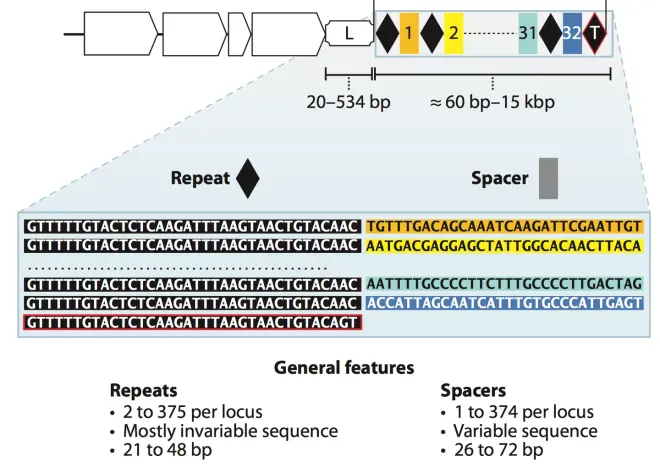
(3) The mechanism of action of CRISPR/Cas system.?
The mechanism of action of CRISPR/Cas system can be divided into two main parts: Adaptation and Interference. The first part, Adaptation, can be further divided into two subparts, namely, Spacer acquisition and CRISPR RNA (crRNA) synthesis and processing. The following explanation of the mechanism is based on Fig.3
1.Adaptation: Spacer acquisition.When phages or foreign genes invade into the bacteria, their protospacer in the genome will be recognized and cut by the cas-related genes in the CRISPR/Cas system. Cas proteins recognize and acquire spacers based on their PAM (Protospacer adjacent motif) sequence downstream of the sequence, which plays a crucial role in spacer acquisition and CRISPR system design in vitro. Different CRISPR/Cas systems have different PAM recognition sequences. When Cas-related proteins select spacers, they will cut their genes and insert them between the leader sequence and the adjacent repeat, forming a new spacer. In this way, the next time the same foreign gene invades, its genome can be cut. There needs to be a repeat in front of the spacer, which is important for forming mature CRISPR RNA later. In order not to affect the reader’s general understanding of the mechanism, how a new repeat sequence is formed in front of the newly inserted spacer.
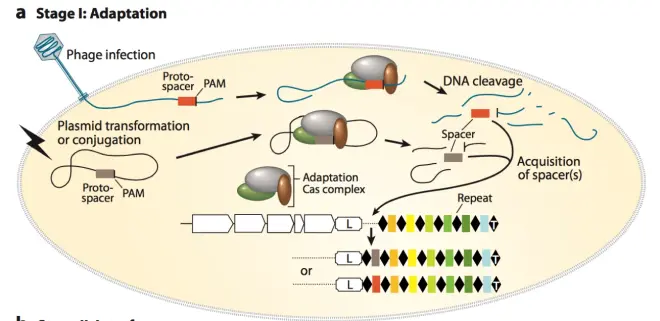
2.Adaptation: CRISPR RNA (crRNA) formation. As mentioned before, the leader sequence has a promoter that can initiate the transcription of the CRISPR array behind it, which is continuous. Therefore, the RNA product transcribed is a long chain, which contains all the spacers and repeats in the CRISPR array, and this long chain RNA is called precursor transcript (pre-crRNA). As shown in Fig.4, the long chain pre-crRNA will be processed and cut by enzymes or Cas-related proteins expressed by housekeeping genes in the bacteria (depending on the difference of CRISPR system), making it mature, single-spacer crRNA. The spacer RNA sequence transcribed is complementary to the target anchored gene, and crRNA can guide Cas-related proteins to cut the genes in the target genome.
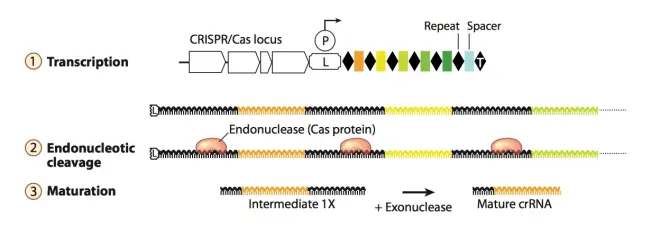
3.Stage II: interference. After the formation of mature single-spacer crRNA, it will form a complex with Cas-related proteins and other RNA components, and crRNA can complementarily pair with genes in the foreign gene, and guide Cas protein or protein complex to cut the foreign gene fragment. As shown in Fig.5 and Fig.6. The complex composed of crRNA and Cas-related proteins is different according to different types of CRISPR systems. In the most commonly used type II system, crRNA will pair with noncoding trans-activating CRISPR RNA (tracrRNA) and then form a complex with Cas9 protein to perform DNA cleavage. This will be introduced later.
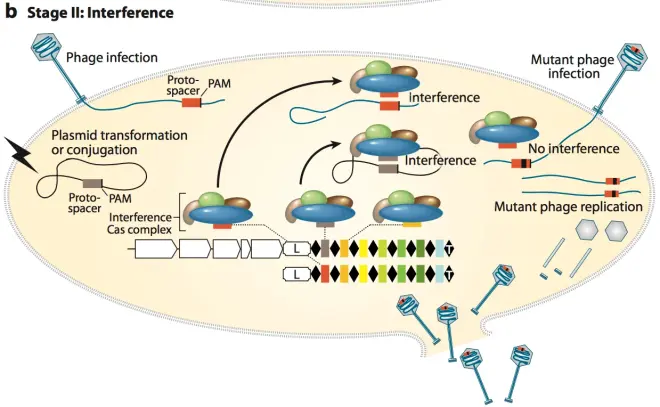
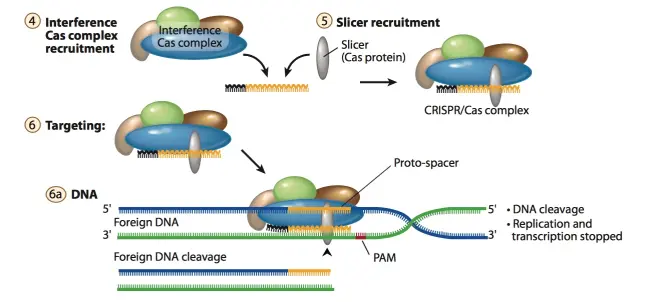
(4) Different CRISPR systems.?
CRISPR/Cas system can be roughly divided into two classes, Class 1 (including type I, III, and IV), and Class 2 (including type II and type V and type VI). In Class 1, the cleavage of foreign genomes requires a large Cas protein complex (composed of more than one Cas protein) and guide RNA. In Class 2, the cleavage of foreign genes only requires a single cleavage protein, such as Cas9 protein in Type II and cpf1 protein in Type V. Fig.7 clearly shows which Cas-related proteins are involved in which stage. When artificially synthesizing Cas system, the most commonly used is Class 2 CRISPR system, because the cleavage of DNA only requires a single Cas9 protein or cpf1 protein, which is very simple and convenient.
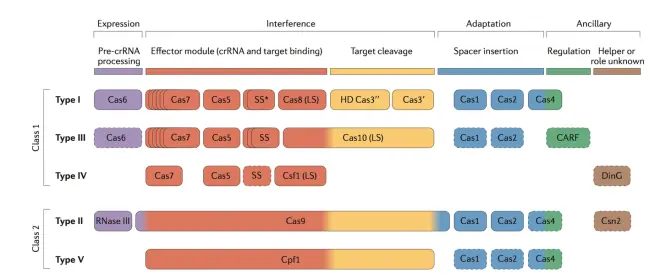
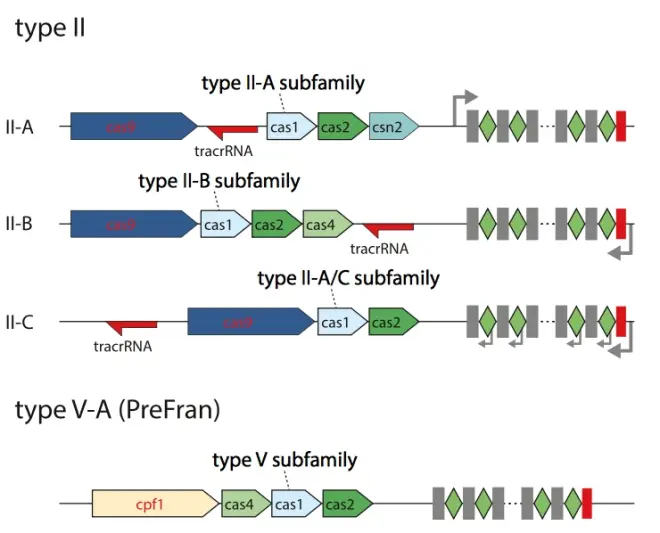
Fig.8 shows the gene structure of CRISPR system in three subtypes of type II and Type V. The functions of each Cas protein can be seen in Fig.7. The tracrRNA was introduced in the previous section. When cleaving DNA, tracrRNA will pair with crRNA to form guided RNA to guide Cas9 to cleave DNA. The detailed structure diagram is shown in Appendix 2.
(5) Type II CRISPR system in vitro synthesis strategy and application principle.
The CRISPR mechanism introduced in Chapter 3 is the mechanism in bacteria, which is the basis for applying CRISPR technology. CRISPR is called a powerful genome editing tool because it is easy to operate and synthesize. As mentioned above, Type II CRISPR system is the most commonly used system. So in this section, I will take type system as an example to introduce the application of CRISPR. First, let’s look at Fig.9, which is a very clear schematic diagram. In practical application, we will first select the gene that needs to be anchored and cut, and the gene selection is based on PAM sequence, which was introduced before. The PAM sequence of type II is NGG. That is to say, on the target cut gene, there must be a PAM sequence after the cut gene fragment, which is necessary for Cas9 recognition and cutting later. After selecting the desired gene fragment, crRNA is the selected fragment. Then tracrRNA is necessary, because it needs to form a special structure with crRNA before it can guide Cas9 protein to cut. As shown in Appendix 2, tracrRNA pairs with crRNA at the repeat gene part, so in practical application, one tracrRNA can pair with different crRNAs (containing the protospacer and repeat sequence of the target gene). And sgRNA (single-guided RNA) is artificially synthesized in vitro, which can guide Cas9 to cut the target gene. Ps: In actual operation, linker loop is not needed. (As shown in Appendix 2), because if linker loop is used, tracrRNA needs to be transcribed for each spacer. If there are multiple spacers in a system, then it can be synthesized according to the gene structure in Fig.8.
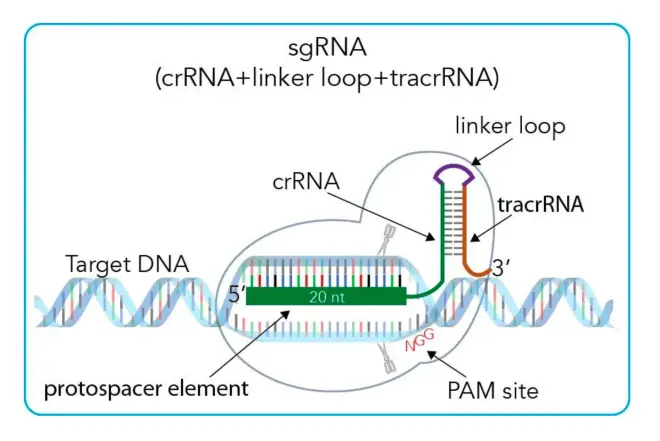
So what to do after cutting the gene? Cas9 protein can induce double-strand breaks in DNA, and Cas9 contains HNH, RuvC-like and PAM sequence interaction domains. HNH and RuvC will each cut one strand of DNA, causing double-strand breaks. As shown in Fig.10. If the HNH and RuvC activity domains are mutated to make them unable to perform the cutting function, then Dead Cas9 (dCas9) will be produced. dCas9 can only tentatively attach to a specific gene position (based on the sequence on sgRNA), but cannot perform the cutting function. dCas9 plays an important role in various applications of CRISPR. Another method is to silence only one of the sites of HNH or RuvC, forming a Cas9 nickase, which can use two different nickases to anchor and single-strand cut the genome, resulting in sticky ends.
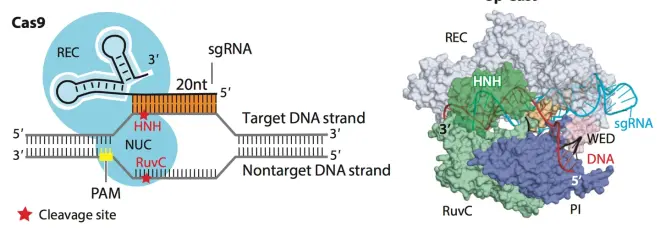
As shown in Fig.10, a single CRISPR system can cause blunt-ended DSB (double-stranded break), and two Cas9 nickase systems can cause sticky-ended break. When the double strand of DNA is cut, there are two ways of repair: The first one is NHEJ, non-homologous end joining. This is a spontaneous SOS repair in the organism, which urgently connects the broken DNA double strand, but this connection method is random, and may cause base insertion, deletion, resulting in reading frame shift. The other one is HDR, homology-directed repair, which provides a small donor DNA fragment with the same end sequence and break sequence. This DNA fragment can undergo homologous recombination with the broken gene, thus forming a purposeful insertion gene, deletion gene and other functions (the red marked fragment is the target insertion fragment). In this way, the directional change, insertion and deletion of genes in the genome can be completed. The third figure in Fig.10 uses dCas9 (dead Cas9). And FokI enzyme is attached to Cas9 protein, so that two dead cas9 can achieve gene localization without cutting, and FokI enzyme performs cutting function. This double-anchoring function will greatly reduce the off-target effect of CRISPR system.
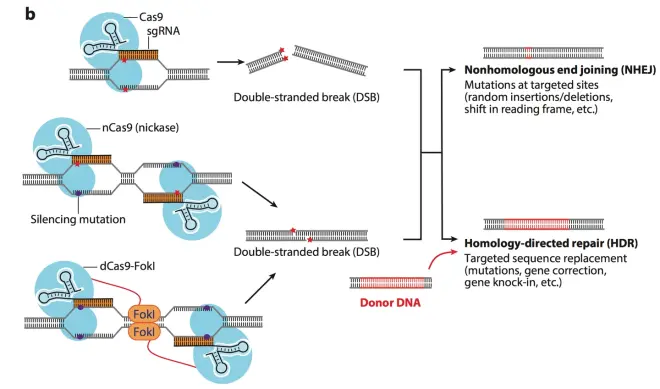

(6) Different applications of CRISPR system.?
The first use of CRISPR system was to cause double-strand breaks in DNA, and to perform qualitative and purposeful gene editing using NHEJ or HDR methods. But soon, CRISPR system was applied to many fields. For example, activating or inhibiting transcription reaction. The following is an introduction based on Fig.12. Figure A shows the normal Cas9 protein and dCas9 protein. The first figure in Figure B fuses the w subunit with the dCas9 protein, so that the Cas9 protein can locate to a specific position, and the w subunit can recruit transcription factors, thus stimulating the transcription process. In the second figure, dcas9 can bind to the downstream of RNA polymerase, blocking the polymerase from continuing transcription and inhibiting transcription. Figure C shows the activation of gene transcription in mammalian cells by fusing VP64 and the inhibition of transcription by fusing KRAB. Figure D is more advanced, compared to Figure C where only one VP64 activation factor is fused, this method fuses a peptide (scFV peptide), which contains VP64, and the peptides can bind to each other, thus greatly increasing the recruitment rate of the activation factor VP64. A dCas9 protein can recruit up to 24 VP64s, greatly increasing the transcription efficiency. CRISPR system has many applications in transcription activation and inhibition, which are not detailed here. Interested readers can read the references below to learn more.
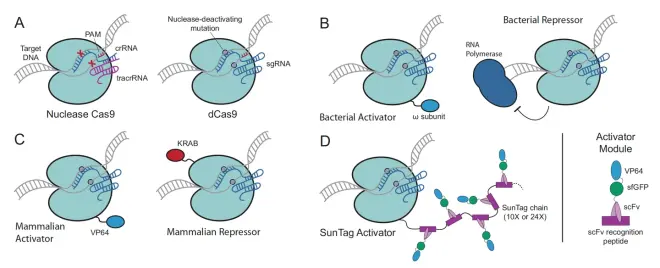
In addition to transcription activation and inhibition, fluorescent proteins can also be fused to dcas9, which can qualitatively bind to specific gene sites by guide RNA guidance, making it easy to observe. This is very useful for observing the expression intensity and expression process of a target gene. (Fig.13)
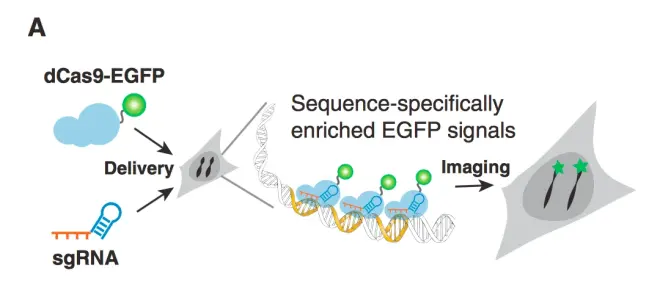

Citation
(1) What is CRISPR? | New Scientist. https://www.newscientist.com/definition/what-is-crispr/.
(2) CRISPR - Wikipedia. https://en.wikipedia.org/wiki/CRISPR.
(3) CRISPR - National Human Genome Research Institute. https://www.genome.gov/genetics-glossary/CRISPR.
(4) CRISPR-?https://baike.baidu.com/item/CRISPR/16691117.
(5) CRISPR - Wikipedia. https://ja.wikipedia.org/wiki/CRISPR.
(6) CRISPR ethics: moral considerations for applications of a .... https://www.ncbi.nlm.nih.gov/pmc/articles/PMC6286228/.
(7) Human genome editing: regulations, risks, and ethical .... https://www.embl.org/news/lab-matters/human-genome-editing-regulations-risks-and-ethical-considerations/.
(8) Human germline editing in the era of CRISPR-Cas: risk and .... https://bmcmedethics.biomedcentral.com/articles/10.1186/s12910-020-00487-1.
(9) Cas9 - Wikipedia. https://en.wikipedia.org/wiki/Cas9.
(10) Cas9 - Wikipedia. https://ja.wikipedia.org/wiki/Cas9.
(11) Cas9 - an overview | ScienceDirect Topics. https://www.sciencedirect.com/topics/biochemistry-genetics-and-molecular-biology/cas9.
(12) What is CRISPR/Cas9? - PMC - National Center for .... https://www.ncbi.nlm.nih.gov/pmc/articles/PMC4975809/.
(13) Cas9 - Wikipedia. https://it.wikipedia.org/wiki/Cas9.


Editor:Linues
Auditor:Philip
Designer:Sally