Catena | 禁牧后溫帶草地土壤碳氮磷儲量的耦合與解耦(長文翻譯)
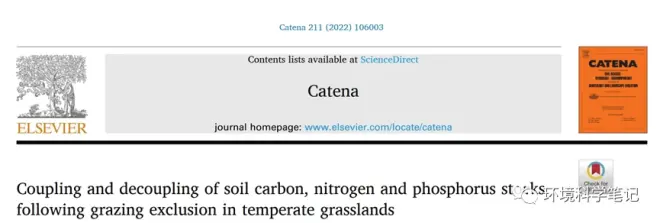
[題目]
Coupling and decoupling of soil carbon, nitrogen and phosphorus stocks following grazing exclusion in temperate grasslands
[作者]
JiweiLi?a?b?c,?Kaibo?Wang?d,?Zhouping?Shangguan?a?b?c,?Lei?Deng?c
[單位]
aState Key Laboratory of Soil Erosion and Dryland Farming on the Loess Plateau, Institute of Soil and Water Conservation, Chinese Academy of Sciences and Ministry of Water Resources, Yangling, Shaanxi 712100, China
bUniversity of Chinese Academy of Sciences, Beijing 100049, China
cInstitute of Soil and Water Conservation, Northwest A&F University, Yangling, Shaanxi 712100, China
dState Key Laboratory of Loess and Quaternary Geology, Institute of Earth Environment, Chinese Academy of Sciences. Xi’an, Shaanxi, 710061, China
Highlights
(1) Soil C and N stocks significantly accumulated following grazing exclusion.
禁牧后土壤碳氮儲量顯著增加。
(2) Soil P stocks tended to decrease after grazed exclusion in temperate grassland.
溫帶草地在禁牧后土壤磷儲量呈下降趨勢。
(3) SOCand TN stocks were decoupled with TP stocks since grazed exclusion.
由于禁牧,SOC和TN庫存與TP庫存解耦。
(4) Soil extracellular enzymes played critical roles in enhancing soil N stocks.
土壤胞外酶在提高土壤氮儲量中起著重要作用。
Abstract
Grazing exclusion is considered a crucial measure for improving ecosystem structure and function, including elements such as soil carbon and nutrients.?禁止放牧被認為是改善生態(tài)系統(tǒng)結構和功能的關鍵措施,包括土壤碳和養(yǎng)分等元素。
However, little is known about the relationships of soil organic C (SOC), total nitrogen (TN), and total phosphorous (TP) stocks during long-term grazing exclusion.?但長期禁牧期間土壤有機碳(SOC)、總氮(TN)和總磷(TP)儲量之間的關系尚不清楚。
This study examined the SOC, TN, and TP stocks after 0–33years of grazing exclusion in temperate grasslands on the Loess Plateau, China.?研究了黃土高原溫帶草地禁牧0–33年后土壤有機碳、全氮和總磷儲量的變化。
The results showed that long-term grazing exclusion significantly increased SOC and TN stocks but declined TP stocks compared to those in grazing grasslands.?結果表明與放牧草地相比,長期禁牧顯著提高了土壤有機碳和全氮儲量,降低了TP儲量;
In addition, grazing exclusion was more favorable for SOC and TN accumulations than TP accretion, and SOC and TN stocks were decoupled from TP stock.?此外,不放牧對土壤有機碳和全氮的積累比TP的積累更有利,且土壤有機碳和全氮的蓄積量與TP的蓄積量呈脫鉤關系。
Overall, changes in SOC stock were determined by plant productivity, and TN stocks were also driven by soil microbial activities (i.e., N-acquiring enzymes).?總體而言,土壤有機碳儲量的變化受植物生產力的影響,而全氮儲量的變化也受土壤微生物活性(即氮獲取酶)的影響。
Nevertheless, plant and microbial characteristics had no effects on TP stocks following grazing exclusion.?然而,植物和微生物特征對放牧后全磷儲量沒有影響。
This study emphasizes the positive effect of grazing exclusion on SOC and TN accumulations, further, it is needed to explore soil phosphorus stabilization mechanisms during grassland restoration after grazing exclusion.?本研究強調了禁牧對土壤有機碳和全氮積累的積極作用,并進一步探討禁牧后草地恢復過程中土壤磷的穩(wěn)定機制。
Keywords
Carbon sequestration(碳固定);Grazing exclusion;Nitrogen sequestration;Temperate grassland;Vegetation restoration
1.Introduction
Long-term overloading and improper grazing can destroy thegrassland ecosystem?function, which causing severe soil erosion, subsurface compaction and degradation in grasslands around the world. Such degradation can gradually impact the quality of animal husbandry and ecological?sustainability, which includes reductions in?soil microbial activity?and resource availability. Consequently, restoration and governance on degraded grasslands are urgently needed, as they have great capacity to preserve a considerable fraction of atmospheric carbon dioxide under appropriate grazing practices. Grazing exclusion is considered a highly effective measure for the restoration of overgrazed grasslands, by improving plant productivity and soil quality. Therefore, obtaining a better understanding of how grazing exclusion influences soil quality is critical for maintaining grassland functionality and sustainability.長期的超載和不當放牧會破壞草地生態(tài)系統(tǒng)功能,造成世界各地草地嚴重的水土流失、地下壓實和退化。這種退化會逐漸影響畜牧業(yè)的質量和生態(tài)可持續(xù)性,包括土壤微生物活性和資源可用性的減少。因此,退化草地的恢復和治理是迫切需要的,因為在適當?shù)姆拍磷龇ㄏ?,它們有很大的能力保存大氣中相當一部分的二氧化碳。禁牧被認為是通過提高植物生產力和土壤質量來恢復過度放牧草地的一種非常有效的措施。因此,更好地理解禁牧如何影響土壤質量對于保持草地功能和可持續(xù)性至關重要。
Soil organic carbon (SOC), total nitrogen (TN), and phosphorus (TP) stocks, and cycling processes are important aspects of sustainable soil quality, and the ecosystem functions of grasslands. Grazing exclusion can directly affect the distribution of SOC, TN, and TP, and exert complex effects onsoil organic matter?(SOM) cycling. However, the responses of SOC, TN, and TP stocks to grazing exclusion have been controversial in grasslands. Some studies have revealed that grazing exclusion leads to increased SOC and nutrients, whereas others have shown that there were negative or even no significant impacts of grazing exclusion on SOC, TN, and TP immobilization in grasslands. These inconsistencies are likely due to variations in grassland types, enclosure durations, grazing history, and factors limiting plant growth; thereby affecting ecosystem SOM cycling in these regions. Thus, the effects of grazing exclusion periods on SOC and nutrients in grasslands remain unclear. Therefore, we aimed to clarify the response and drivers of SOC, TN, and TP stocks and to scientifically explore a grassland management strategy for improving the production and ecological functions of grasslands through grazing exclusion.?土壤有機碳(SOC)、全氮(TN)和全磷(TP))儲量及其循環(huán)過程是影響草地土壤可持續(xù)質量和生態(tài)系統(tǒng)功能的重要方面。禁牧直接影響土壤有機碳、全氮和全磷的分布,并對土壤有機質循環(huán)產生復雜的影響。然而,草地SOC、TN和TP儲量對禁牧的響應一直存在爭議。一些研究表明,禁牧導致草地SOC和養(yǎng)分增加,而另一些研究表明,禁牧對草地SOC、TN和TP固定具有負效應甚至不顯著的影響。這些不一致性可能是由于草地類型、封育時間、放牧歷史和限制植物生長的因素的差異;從而影響這些區(qū)域的生態(tài)系統(tǒng)有機質循環(huán)。因此,禁牧期對草地有機碳和養(yǎng)分的影響尚不清楚。因此,本研究旨在闡明SOC、TN和TP儲量的響應及其驅動因素,科學探索通過禁牧提高草地生產和生態(tài)功能的草地管理策略。
Ecological restoration?via grazing exclusion can alter the distributions of SOC, TN, and TP in grassland ecosystems. The persistence and stabilization of SOC, TN, and TP stocks are altered by biotic and?abiotic factors, including vegetation characteristics, aggregate physical properties, and microbial?nutrient use efficiency. In general, greater plant productivity and microbial biomass represent greater root surface areas and higher SOC and TN stocks. Previous studies have focused on the impact of biological and abiotic factors, such as grazing exclusion period, soil physicochemical variables,?plant characteristics, and?microorganismson the variations in TN and SOC sequestration in grassland ecosystems. Nevertheless, most of these studies have only compared single un-grazed grasslands against grazed grasslands and focused on the single periods of exclusion. In addition, these studies have mostly ignored the combined impacts of biotic and abiotic variables on SOC, TN, and TP stocks. Therefore, we aimed to clarify the proportionate contributions of microbial, plant, and edaphic variables on TN, TP, and SOC stocks; and to scientifically explore a grassland management strategy for improving the ecological functions of grasslands through grazing exclusion practices.?通過放牧進行生態(tài)恢復可以改變草地生態(tài)系統(tǒng)SOC、TN和TP的分布。植被特征、團聚體物理性質和微生物養(yǎng)分利用效率等生物和非生物因素影響著土壤SOC、TN和TP儲量的持久性和穩(wěn)定性??傮w而言,較高的植物生產力和微生物生物量代表較大的根系表面積和較高的SOC和TN儲量。以往的研究主要集中在放牧封育期、土壤理化變量、植物特征和微生物等生物和非生物因素對草地生態(tài)系統(tǒng)全氮和有機碳固存變化的影響。然而,這些研究大多只比較了單一的未放牧草地和放牧草地,并集中在單一的排除時期。此外,這些研究大多忽略了生物和非生物變量對SOC、TN和TP儲量的聯(lián)合影響。因此,本研究旨在闡明微生物、植物和土壤變量對全氮、全磷和有機碳儲量的比例貢獻;科學探索通過禁牧改善草地生態(tài)功能的草地管理策略。
Grazing exclusion facilitatesSOM formation?and stability by altering both the quality and quantity of litter and root input, and consequently, indirectly shifts in SOC, TN, and TP dynamics. To our knowledge, most studies have principally focused on comparing SOC stocks of grazed and un-grazed lands; however, there are limited studies on nutrient stocks (especially TP stocks). In addition, soil TP stocks have been mainly derived from rock weathering, whereas soil TN stocks are influenced by?nitrogen fixation, deposition input, and?inorganic nitrogen?leaching. Previous studies have proposed that soil TN and TP dynamics may strictly limit the soil carbon (C) accumulation during vegetation?recolonization?in natural?terrestrial ecosystems. In general, SOC accumulation is coupled to TN because of its influence on SOM decomposition. However, experiments aimed at investigating changes in soil TN and TP stocks have not been attempted, and few studies have paid close attention to the associations between SOC, TN, and TP stocks following grazing exclusion. Therefore, it is necessary to evaluate the responses of C and nutrient stocks to grazing exclusion.?禁牧通過改變凋落物和根系輸入的質量和數(shù)量,促進SOM的形成和穩(wěn)定,從而間接改變SOC、TN和TP的動態(tài)。據(jù)我們所知,大多數(shù)研究主要集中在比較放牧和未放牧土地的有機碳儲量;然而,對營養(yǎng)儲量(特別是總磷儲量)的研究有限。此外,土壤TP儲量主要來源于巖石風化,而土壤TN儲量受氮固定、沉積輸入和無機氮淋溶的影響。已有研究表明,在自然陸地生態(tài)系統(tǒng)中,土壤TN和TP動態(tài)可能嚴格限制了植被再定植過程中土壤碳(C)的積累??傮w上,SOC的積累與TN的耦合作用影響SOM的分解。然而,目前還沒有針對土壤全氮和全磷儲量變化的試驗,而且很少有研究關注放牧后土壤有機碳、全氮和全磷儲量之間的關系。因此,有必要評估碳儲量和養(yǎng)分儲量對禁牧的響應。
To recover ecosystem function in theLoess?Plateau region, the Chinese government has implemented the ‘Returning?Grazing Land?to Grassland’ project, which prohibits grazing in many grasslands through grazing exclusion. Vegetation characteristics, soil physical and chemical properties, and?microbial activities?may be altered through grazing exclusion. However, there is limited information on the effects of long-term grazing exclusion strategies on SOC, TN, and TP stocks. In addition, limited information is available about which microbial, plant, and edaphic variables have the impacts on the stocks of SOC, TN, and TP. To address these knowledge gaps, this study sampled soils that represent a natural successional sequence following grazing exclusion for 0, 8, 18, 26, and 33?years on the Chinese Loess Plateau; to (1) examine how SOC, TN, and TP stocks responded, and (2) to identify the key microbial, plant, and edaphic variables that affect SOC, TN, and TP stocks after grazing exclusion.?為了恢復黃土高原地區(qū)的生態(tài)系統(tǒng)功能,中國政府實施了“退牧還草”工程,在許多草原上實行禁牧。植被特征、土壤理化性質和微生物活動可能會通過放牧被改變。然而,目前關于長期禁牧策略對SOC、TN和TP儲量的影響的信息有限。此外,關于哪些微生物、植物和土壤變量對SOC、TN和TP儲量有影響的信息有限。為了填補這些知識空白,本研究以黃土高原放牧0、8、18、26和33年的自然演替序列為樣本;(1)研究SOC、TN和TP儲量的響應;(2)識別放牧后影響SOC、TN和TP儲量的關鍵微生物、植物和土壤變量。
2.Materials and methods
2.1.Study area and field sampling
The study area was the Yunwu Mountain grassland, Ningxia Province, China, located northeast of Guyuan and in the middle of theLoess?Plateau (36°10′–36°17′N, 109°16′–109°24′E; altitude, 1700–2148?m above sea level). Since 1980, the government has implemented several strict measures to exclude livestock and farmers for ecological restoration purposes in the Yunwu Mountain region. The area thus provides a range of exclusion ages for study. The annual average temperature is 7?°C, and the annual average precipitation is 425?mm, 60%–75% of the precipitation occurs in summer between July and September, and atmospheric precipitation in the main water supply. The area has a typical temperate semi-arid climate, and the vegetation is typical of temperate grassland. The soil type in the study area was aeolian soil (silt loam), and the dominant?plant species?were?Stipa grandis,?Potentilla?acaulis,?Artemisia capillaris, and?Thymus mongolicus.
In the experimental area, achronosequence?of five grasslands with different?grazing exclusion periods (0, 8, 18, 26, and 33?years)?was selected to investigate when the aboveground biomass peaked based on measurements in?2019. Grazed grassland was used as a control (GE0), consisting of an unfenced free-grazing community. All sites had similar soil types and?geographical characteristics?(Table S1). At each site, six plots (20?m?×?20?m) were established at least 10?m apart as independent replicates. Within each plot, three quadrats (1?m?×?1?m) were randomly established, and in each quadrat, plant community coverage (PCC), plant community height (PCH), litter biomass (LB), belowground biomass (BB), and aboveground biomass (AB) were measured as described by?Deng et al. (2014). For each of the five grassland ages, twenty upper mineral soil cores (0–10 cm) were randomly collected from each layer in each plot and mixed thoroughly to produce a composite sample using a 2-mm sieve after removing the aboveground tissues. Then, the soil samples were placed in a portable cooler box and transported to the laboratory for further measurement. The soil samples were subsequently divided? into two parts: one part was applied to measure soil extracellular?enzyme activities?and microbial biomass at 4?°C for up to 2?weeks, and the other part was used to text soil physical and chemistry properties with air-drying at 25?°C.
2.2.Soil physical and chemistry properties
Soil bulk density?(BD, g cm?3) were determined by the cutting ring method.Soil organic carbon?(SOC) and?total nitrogen(TN) were determined using the calorific boiling and?Kjeldahl method, respectively (Nelson and Sommers, 1983). A suspension in a 1: 2.5:1 ratio of soil to water was applied to measure?soil pH?by a?pH?meter (Mettler Toledo, USA). Soil moisture content (SW, %) was determined gravimetrically by weight loss after oven-drying 100?g of fresh soil at 105?°C for 48?h. Soil?particle size distribution?was analyzed using a Mastersizer 2000 laser sizer (Malvern Instruments Ltd., Malvern, UK), and was, then, divided into sand, silt, and clay.?Dissolved organic carbon?(DOC) concentrations were measured using a?total organic carbon?(TOC) analyzer.?Soil available N(SAN) concentration were analysed by the continuous alkali-hydrolyzed reduction diffusing (Stanford, 1982). The contents of?soil available phosphorus(SAP) and?total phosphorus(TP) were measured by an?ultraviolet spectrophotometer?(Hitachi UV2300) after extracted with 0.5?M NaHCO3?and H2SO4-HClO4, respectively.
2.3. Soil extracellular enzyme activities and microbial biomass
The activities of?threehydrolytic enzymes?(β-1,4-glucosidase, BG;?β-1,4-N-acetylglucosaminidase, NAG;?alkaline phosphatase, ALP) were assayed. The activities of BG for microbial C-acquisition, NAG for microbial N-acquisition, and ALP for microbial P-acquiring. Fresh soil (eight duplicate) was dispensed into 96-well?microplates?with buffer solution. Fluorescence were assayed by a microplate reader with 450-nm emission and 365-nm excitation filte after the solution incubated at 25?°C for 4?h in the dark. The activities of soil extracellular enzyme were expressed as μmol g?1?h?1. The chloroform?fumigation extraction?method was used to determine the?soil microbial biomass?carbon?(MBC) and?nitrogen?(MBN), extracting using K2SO4?(100?mL 0.5?mol L-1) (Brookes et al., 1985). The extractions were measured for MBC and MBN using a Total Organic C/N analyzer. Conversion factors used to calculate MBC and MBN were 0.45 and 0.54 by?Brookes et al. (1985), respectively.?Microbial biomass phosphorus?(MBP) was extracted by 0.5?M NaHCO3, using the fumigation extraction method described by?Brookes et al. (1982). The experimental conversion factor for calculating MBP was 0.40 (Brookes et al., 1982).
2.4.Estimation of the TN, TP, and SOC stocks
TN, TP, and SOC stocks were calculate by the following equations (Li et al., 2020a):
whereX?denotes the SOC, TN, or TP stocks after grazing exclusion, TN is the soil total N content (g kg?1), BD is the soil bulk density (kg m?3), SOC is the soil organic C content (g kg?1), TP is the total phosphorus content, and D is the soil layer thickness (cm).
2.5.Calculation of TN, TP, and SOC sequestration
We used the following equations to calculate SOC, TN, and TP sequestrations (Deng et al., 2017,Li et al., 2012):

where?△X denote the variations in the stocks of TN, TP, or SOC after grazing exclusion, respectively;?XGEt0?are the stocks of TN, TP, or SOC in the grazing grassland; and XGEt?express stocks of SOC, TN, or TP at the site after grazing exclusion for t years, respectively.
2.6.Estimation of the change rates of SOC, TN, and TPstocks
We applied the following equations to estimate the rates of relative change in the stocks of TN, TP, or SOC (Deng et al., 2016,Li et al., 2012,?Li et al., 2020a):

where stockt0is the TN, TP, or SOC stocks in the grazing grassland, Δt denotes the time (years) since after grazing exclusion, and ΔX express the change in the stocks of TN, TP, or SOC after grazing exclusion.
2.7.Statistical analysis
Linear mixed-effects models (LMM) were used to test the effects of grazing exclusion time, soil depth, and their interaction on each variable using the ‘nlme’ function in R (version 4.0). When analyzing the SOC, TN, or TP stocks andsoil physicochemical properties, ‘soil depth’, ‘grazing exclusion’, and their ‘interaction’ were used as the fixed factors, whereas ‘plot’ was used as a random factor. Redundancy analysis (RDA) was also used to determine the effects of plant and soil variables on the SOC, TN, and TP stocks with the ‘vegan’ package in R (version 4.0). To elucidate the relative influences of microbial, edaphic and vegetation variables on the SOC, TN, and TP stocks, multivariable linear regression analysis (MLRA) was performed the relative importance of plant, soil, and microbial variables to explain the SOC, TN, or TP stocks. Before the analysis, variables with high variance inflation factors (VIF?>?20) were eliminated from the model to exclude collinearity. However, no factor was found to control TP stocks (R2?=?0.11,?P?=?0.40).?Structural equation modeling?(SEM) frameworks were then applied to reveal both the indirect and direct impacts of the driving variables on the TN, TP, and SOC stocks in AMOS 23.0.
3.Results
3.1.Variations in TN, TP, and SOC stocks since grazing exclusion
Compared with stocks in the free-grazing site, grazing exclusion significantly increased SOC stocks (SOCS) and TN stocks (TNS) but decreased TP stocks (TPS,P?<?0.05). In the 0–20?cm layer, 26 and 33?years of grazing exclusion significantly increased SOCS by 216.8% and 207.0%, respectively (Fig. 1a); whereas in the initial 18?years after grazing exclusion, no significant differences in SOCS were found in the 40–60?cm layer (Fig. 1a). After 33?years of grazing exclusion, TNS was significantly higher than in the initial 26?years following grazing exclusion in the 0–20?cm layer (Fig. 1b), but there was little difference in the TNS after grazing exclusion in the 40–60?cm layers. In contrast, grazing exclusion resulted in a significantly lower TPS in the 0–40?cm soil layer (P?<?0.05) (Fig. 1c) than in free-grazing sites.
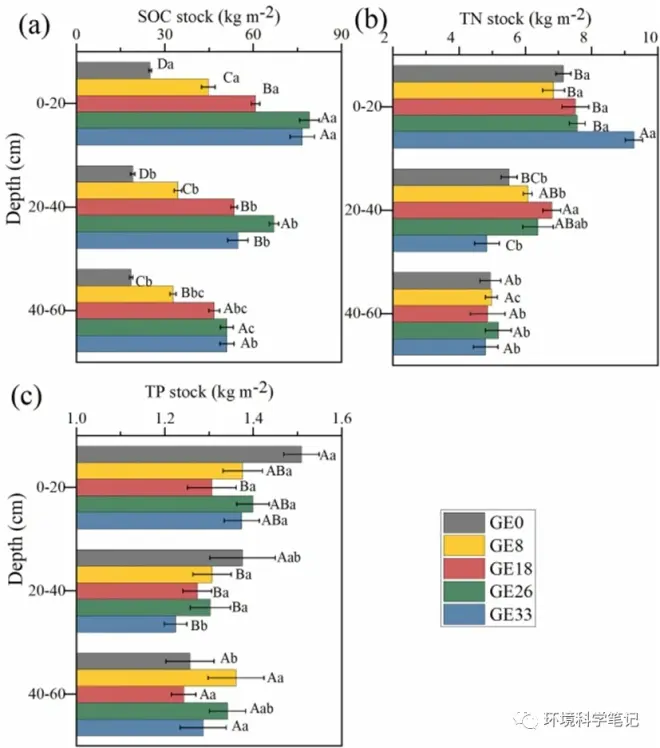
Fig. 1.Effects of grazing exclusion on the distribution of?soil organic carbon?(SOC, a), total nitrogen (TN, b), and total phosphorus (TP, c) stocks at 0–60 soil layer.
The values of ΔSOCS at the depths of 0–60cm significantly increased after 8?years grazing exclusion, but ΔSOCS presented no significant differences after 26 and 18?years of grazing exclusion in the 0–60?cm layers (Fig. 2a). Grazing exclusion for 33?years significantly increased ΔTNS in the 0–20?cm layer, but significantly decreased ΔTNS in the 20–40?cm layer (Fig. 2b). Soil TPS showed no significant differences after grazing exclusion (Fig. 1c). The rates of relative changes in SOCS and TNS decreased continuously across the 33-year period of grazing exclusion (Fig. 2d and e). The values of ΔTNS and ΔTPS showed no significant changes in the 0–60?cm layer (Fig. 2e and f).
Fig. 2. Effects of grazing exclusion on the sequestrations (a-c) and accumulation rates (d-f) of soil organic carbon (SOC, a), total nitrogen (TN, b), and total phosphorus (TP, c) stocks at 0–60 soil layer.
3.2.Relationships of soil TN, TP, and SOC changes since grazing exclusion
The relationships among SOC, TN, and TP pools exhibited diverse trends after grazing exclusion (Fig. 3). Significant relationships were found between the shifts in the SOC and TN contents (R2=?0.44,?P?<?0.001, y?=?8.53x-1.62;?Fig. 2a), SOC and TP contents (R2?=?0.38,?P?<?0.001, y?=?90.86x-29.37;?Fig. 2b), and TN and TP contents (R2?=?0.20,?P?<?0.001, y?=?5.01x-0.21;?Fig. 2c). However, there were no significant correlations between the changes in the stocks and sequestrations of SOC, TN, and TP, except for a highly correlated relationship between changes in SOCS and TNS (R2?=?0.17,?P?<?0.001, y?=?5.35x?+?14.58;?Fig. 2d-i).
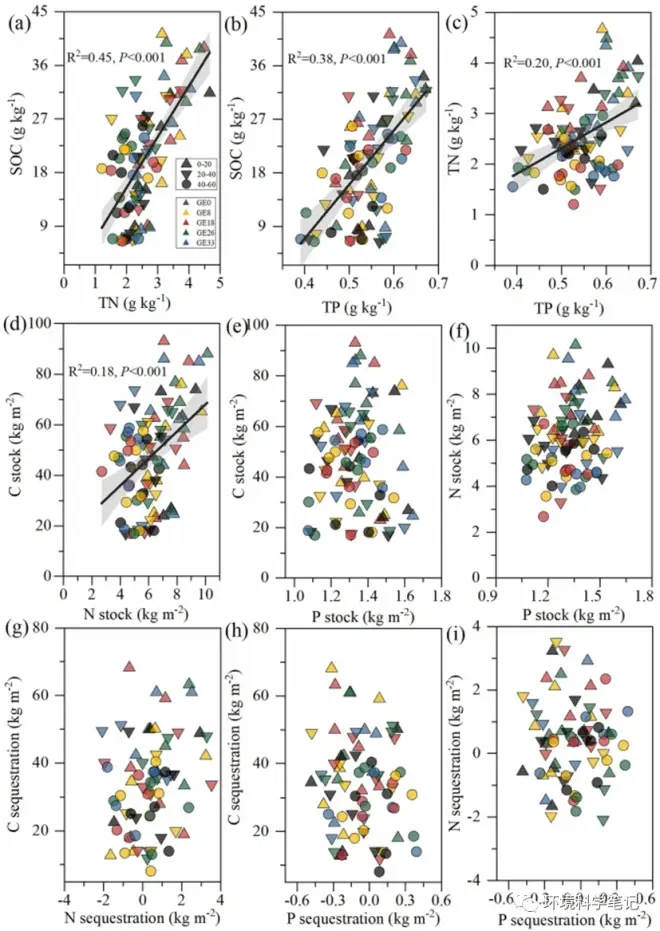
Fig. 3.Regression relationships of?SOC, TN, and TP in contents, stocks, and sequestrations after grazing exclusion. Gray lines indicate 95% confidence intervals, while black lines are the linear model fits of these linear models between soil SOC and TN, TP in sequestrations and accumulation rates. Note: SOC, soil organic carbon; TN, total nitrogen; TP, total phosphorus.
3.3.Factors correlated with the TN, TP, and SOC stocks
The RDA analysis revealed that the RDA1 and RDA2 axes explained 95.35% and 4.59%, respectively, of the variation in the SOCS, TNS, and TPS in the 0–60cm layer (Fig. 4). SOCS was positively correlated with most of the vegetation characteristics, including PCC, PCH, AB, LB, and MBC. In contrast, the TPS were not associated with any environmental factors (Fig. 5c). The results of the MLRM showed that the environmental factors explained 82.1% of the variability in SOCS (Fig. 5a) and 58.9% of the variability in TNS (Fig. 5b). The variations in SOCS were best explained by PCC, LB and DOC (Fig. 5a), whereas NAG, AB, and BB had strong influences on the variation in TNS (Fig. 5b). Furthermore, none of the measured factors were found to control TPS.
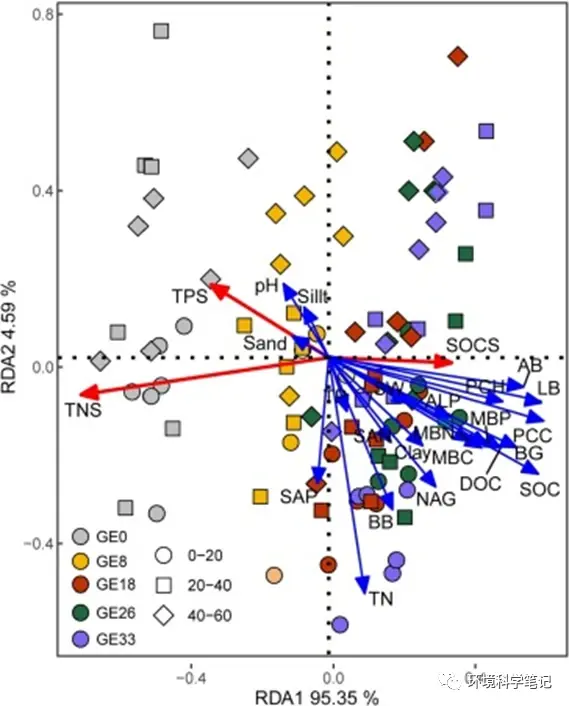
Fig. 4.Redundancy analysis (RDA) ordination diagram for?SOC, TN, and TP stocks with environmental variables at 0–60?cm soil layer as affected by grazing exclusion. Note: SOC, soil organic carbon; TN, total nitrogen; TP, total phosphorus;?AB, aboveground biomass;?BB, belowground biomass; PCC, plant community coverage; LB, litter biomass; PCH, plant community height; pH,?soil pH; BD, bulk density; SW, soil water;?DOC, dissolved organic carbon; SAN, available nitrogen; SAP, available phosphorus;?MBC, microbial biomass carbon; MBN, microbial biomass nitrogen; MBP, microbial biomass phosphorous; BG, β-1,4-glucosidase; NAG, β-1,4-N-acetylglucosaminidase; ALP, alkaline?phosphatase.
Fig. 5.Effects of plant, soil, and microbial properties on SOC (a) and TN (b) stocks from multivariable linear regression model and the Pearson correlation?matrix analysis?among SOC, TN, and TP stocks and environmental variables at 0–60?cm soil layer as affected by grazing exclusion (c). No factor was found to control TP stock (R2?=?0.11,?P?=?0.40). Note: Red indicates positive correlations, and blue indicates negative with environmental variables in Pearson correlation matrix analysis. SOCS, soil organic carbon stock; TNS, total nitrogen stock; TPS, total phosphorus stock; SOC, soil organic carbon; TN, total nitrogen; TP, total phosphorus;?AB, aboveground biomass;?BB, belowground biomass; PCC, plant community coverage; LB, litter biomass; PCH, plant community height; pH,?soil pH; BD, bulk density; SW, soil water;?DOC, dissolved organic carbon; SAN, available nitrogen; SAP, available phosphorus;?MBC, microbial biomass carbon; MBN, microbial biomass nitrogen; MBP, microbial biomass phosphorous; BG, β-1,4-glucosidase; NAG, β-1,4-N-acetylglucosaminidase; ALP, alkaline?phosphatase.
The SEM models showed that the critical factors explained 75% and 57% of the variation in the SOCS (Fig. 6a) and TNS (Fig. 6b), respectively. Grazing exclusion significantly affected the effect of PCC and LB on SOCS variation (Fig. 6a), and LB and DOC directly affected SOCS (Fig. 6a). Moreover, grazing exclusion exerted significant positive impacts on BB and NAG, but a negative effect on AB in affecting TNS variation (Fig. 6b). BB and NAG showed remarkably favorable impacts on the TNS (Fig. 6b), whereas it had a significantly negative and direct impact on the TNS variation. Additionally, the total standardized effects of grazing exclusion, PCC, LB, and DOC on SOCS were 0.82, 0.45, 0.36, and 0.34, respectively (Fig. 6c), and the effects of grazing exclusion, BB, AB and NAG on TNS were 0.13, 0.67, ?0.42 and 0.48, respectively (Fig. 6d).
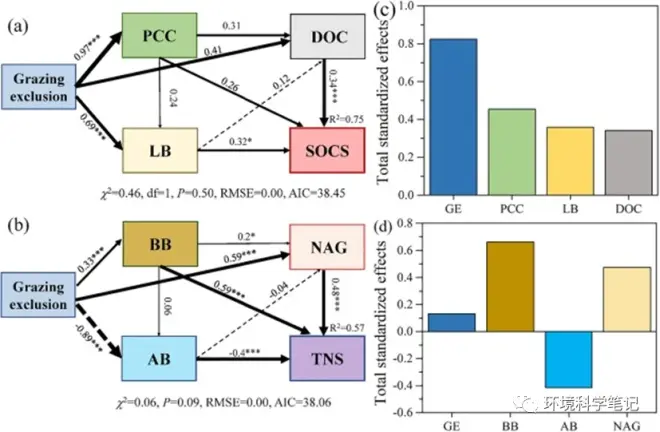
Fig. 6.The structural equation model (SEM) examining the effects of key factors on the contributions of the direct and indirect regulatory pathways of SOC and TN stocks at 0–60 soil layer (no factor was found to control TP stock). Note: SOCS, soil organic carbon stock; TNS, total nitrogen stock; TP, total phosphorus; AB, aboveground biomass; BB, belowground biomass; PCC, plant community coverage; LB, litter biomass; PCH, plant community height; pH, soil pH; BD, bulk density; SW, soil water; DOC, dissolved organic carbon; SAN, available nitrogen; SAP, available phosphorus; MBC, microbial biomass carbon; MBN, microbial biomass nitrogen; MBP, microbial biomass phosphorous; BG, β-1,4-glucosidase; NAG, β-1,4-N-acetylglucosaminidase; ALP, alkaline phosphatase.
4.Discussion
4.1.Effects of grazing exclusion on SOC, TN, and TP stocks
Changes in the accumulation of SOC, TN, and TP following grazing exclusion play critical roles in estimating land recovery in degraded grasslands, and forecasting the effects of grassland recovery on global climate warming. However, the dynamics of SOC, TN, and TP cycles following grazing exclusion are rather complex, and few studies have focused on TP accumulation in arid and semi-arid regions. Based on our results, grazing exclusion (8–33years) significantly increased SOC stock and sequestration in the 0–60?cm soil profile (Fig. 1a). The increases observed in our study may have been caused by the increased inputs of plant and litter biomass after the grazing exclusion (Table S1). Generally, grazing exclusion reduces?soil compaction?by livestock trampling and improves soil?water holding capacity?and plant productivity, thereby leading to higher soil C stocks. However, some studies have reported that grazing exclusion has a negative effect on SOC accumulation. This inconsistency may be due to the shorter recovery periods, low precipitation, and extreme cold conditions in the study areas, which limit vegetation development.?禁牧后土壤有機碳(SOC)、總氮(TN)和總磷(TP)積累的變化對評估退化草地土地恢復、預測草地恢復對全球氣候變暖的影響具有重要意義。然而,禁牧后土壤有機碳、全氮和總磷的動態(tài)變化較為復雜,對干旱半干旱區(qū)土壤總磷積累的研究較少。根據(jù)我們的結果,不放牧(8-33年)顯著增加了0-60 cm土壤剖面上的有機碳存量和固相量(圖1a)。在我們的研究中觀察到的增加可能是由于排除放牧后植物和凋落物生物量投入的增加造成的(表S1)。一般來說,禁牧減少了家畜踩踏造成的土壤壓實,提高了土壤持水能力和植物生產力,從而提高了土壤碳儲量。但也有研究表明,禁牧對土壤有機碳積累有負面影響。這種不一致可能是由于研究地區(qū)的恢復周期較短、降水較少和極端寒冷的條件限制了植被的生長。
Compared to those on SOCS, there are relatively few reports on soil TNS and TPS following grazing exclusion. In our study, grazing exclusion decreased TP stock (Fig. 1c) and sequestration (Fig. 2c), whereas grazing exclusion for 33?years significantly increased TN stock (Fig. 1b) and sequestration (Fig. 2b) within the 0–20?cm soil layer. Generally,?terrestrial ecosystem?nitrogen (N) pools rely on atmospheric N deposition, plant N fixation or both. The high soil TNS in the grazing exclusion sites was mainly linked to?plant litter?return. Importantly, 33?years of grazing exclusion can provide sufficient N stocks, which may be related to the N-fixing species?plant species?that fix N from the atmosphere. In addition, the declines in soil TPS due to grazing exclusion may be related to?soil leaching?and plant consumption. As the new phosphorus (P) is mainly derived from rock weathering, grazing exclusion leads to high soil P loss through the promotion of plant cover and soil moisture recovery. Our results showed a significant correlation between soil P content and soil water (Fig. 5c), suggesting that soil P loss is to some extent linked to water erosion. Furthermore, soil TNS and TPS showed no significant differences in the 20–60?cm layer with grazing exclusion (Fig. 1b and c), indicating that deeper soils such as the 20–60?cm layer are less sensitive to grazing exclusion than?topsoil?layers, and potentially, that extended restoration periods are needed for noticeable accumulation of soil TNS and TPS to occur in the?subsoil.?與SOCS相比,禁牧后土壤TNS和TPS的研究報道相對較少。在我們的研究中,禁牧降低了全氮儲量(圖1c)和固存量(圖2c),而33年的禁牧顯著增加了0-20 cm土層的全氮儲量(圖1b)和固存量(圖2b)。通常,陸地生態(tài)系統(tǒng)氮庫依賴于大氣氮沉降、植物氮固定或兩者兼有。禁牧地高土壤TNS主要與植物凋落物歸還有關。重要的是,33年的禁牧可以提供足夠的氮儲量,這可能與固定氮的植物物種從大氣中固定氮有關。此外,由于禁牧導致的土壤TPS下降可能與土壤淋洗和植物消耗有關。由于新磷主要來源于巖石風化,禁牧通過促進植物覆蓋和土壤水分恢復導致土壤磷流失。我們的結果表明,土壤磷含量與土壤水分之間存在顯著的相關性(圖5c),表明土壤磷的流失在一定程度上與水土流失有關。此外,在禁牧條件下,20-60 cm土層的土壤總養(yǎng)分和總養(yǎng)分利用率沒有顯著差異(圖1b和c),這表明較深層的土壤(如20-60 cm土層)對禁牧的敏感性低于表層土壤,而且可能需要延長恢復期才能使土壤總養(yǎng)分和總養(yǎng)分利用率顯著地積累到下層土壤中。
4.2.Interactions of SOC, TN, and TP accumulations after grazing exclusion
The interactions of SOC, TN, and TP play key roles in terrestrial ecosystemsustainability. Grazing exclusion can promote vegetation succession and?recolonization, leading to redistribution of SOC, TN and TP. We found that the contents of SOC, TN, and TP showed a positive correlation following grazing exclusion (Fig. 3a-c). These findings are consistent with those of the previous studies. There are limited results regarding the correlations between soil TP changes and SOC and TN accumulation following grazing exclusion. Interestingly, our results only found a close relationship between SOCS and TNS (Fig. 3d), but not between SOCS and TPS, or TNS and TPS, as well as their rates of accumulation (Fig. 3e-i). These results indicate that SOC, TN, and TP sequestration were decoupled after grazing exclusion. In general, grazing exclusion increases SOC and TN stocks because there are sufficient inputs of?plant residue?in the?grassland ecosystem; and because fresh P is mainly derived from rock weathering, it might not adapt the elevated SOC and TN in soil. As the SAP from soil resources has been depleted, plants and?soil microorganisms?may acquire more P to meet their growth. What 's more, resource P deficiency might aggravate the competition between vegetation and soil microorganisms under high soil moisture levels following grazing exclusion. Therefore, soil TPS would be decoupled from soil C and N stocks. SOC、TN和TP的交互作用對陸地生態(tài)系統(tǒng)的可持續(xù)性具有關鍵作用。禁牧可促進植被演替和再定植,導致SOC、TN和TP的再分布。結果表明:禁牧后,土壤SOC、TN、TP含量呈顯著正相關(圖3a-c)。這些發(fā)現(xiàn)與之前的研究結果一致。目前關于禁牧后土壤TP變化與SOC和TN積累的相關性研究結果有限。有趣的是,我們的結果只發(fā)現(xiàn)了SOCS和TNS之間的密切關系(圖3d),而沒有發(fā)現(xiàn)SOCS和TPS之間,或者TNS和TPS之間,以及它們的累積速率之間的密切關系(圖3e-i)。綜上所述,禁牧后,土壤SOC、TN和TP固存量均出現(xiàn)去耦。總體而言,禁牧增加了草地生態(tài)系統(tǒng)中充足的植物殘渣輸入,增加了SOC和TN儲量;新鮮P主要來源于巖石風化,可能不適應土壤SOC和TN的升高。隨著土壤資源的枯竭,植物和土壤微生物可能會獲得更多的磷以滿足其生長。此外,資源磷缺乏可能會加劇放牧后高水分條件下植被與土壤微生物之間的競爭。因此,土壤TPS將與土壤碳和氮儲量脫鉤。
4.3.Factors controlling SOC, TN, and TP stocks after grazing exclusion
Grazing exclusion increased vegetation cover, plant community height, and plant biomass as a result of decreased livestock trampling and BD. Thus, soil microorganisms may increase their investments in extracellular enzymes in response to increases in litter andrhizosphere?inputs after grazing exclusion. Our results revealed that SOCS was correlated with most of the measured plant, soil, and microbial variables (Fig. 5a), suggesting that it is sensitive to the environmental changes following grazing exclusion. Among them, plant cover had the greatest effect on SOCS (Fig. 5a and?Fig. 6c), indicating that vegetation community characteristics play critical roles in soil C accumulation. First, grazing exclusion reduces consumption and trampling of plants by livestock and promotes the recovery of the soil microenvironment (e.g., soil temperature, BD, and moisture) and vegetation structure. Second, changes in the microenvironment facilitate the decomposition of the continuous input of litter from various types of vegetation, which acts as a major source of soil C accumulation. It has been demonstrated that after vegetation cover, LB is the second most important factor affecting soil C accumulation (Fig. 5a and?Fig. 6c). Third, surface litter and humus decomposition can alter the community structure and activities of soil microbes, contributing to the formation of?macroaggregates. Soil aggregates can protect?SOM?through a wide variety of pathways, from incorporation to adsorption, resulting in SOC accumulation. Finally, increments in the vegetation cover can alleviate SOC loss by hydraulic and wind erosion. The complex impacts of these factors result in increases in SOCS and are accompanied by extensions in vegetation cover.?禁牧增加了植被蓋度、植物群落高度和植物生物量。因此,土壤微生物可能增加了對細胞外酶的投資,以響應禁牧后枯落物和根際輸入的增加。我們的結果表明,SOCS與大部分測量的植物、土壤和微生物變量相關(圖5a),表明它對禁牧后的環(huán)境變化很敏感。其中,植物覆蓋度對SOCS的影響最大(圖5a和圖6c),說明植被群落特征對土壤碳積累起關鍵作用。首先,禁牧減少了牲畜對植物的消耗和踐踏,促進了土壤微環(huán)境(如土壤溫度、BD和水分)和植被結構的恢復。其次,微環(huán)境的變化促進了各類植被連續(xù)輸入的凋落物的分解,是土壤碳積累的主要來源。研究表明,在植被覆蓋之后,LB是影響土壤C積累的第二重要因素(圖5a和圖6c)。第三,地表枯落物和腐殖質分解可以改變土壤微生物的群落結構和活性,促進大團聚體的形成。土壤團聚體可以通過多種途徑保護SOM,從并入到吸附,導致SOC積累。最后,植被覆蓋度的增加可以緩解水蝕和風蝕造成的SOC損失。這些因素的復雜影響導致了SOCS的增加,并伴隨著植被覆蓋的擴展。
In contrast to SOCS, belowground biomass and NAG exhibited strong impacts on TNS (Fig. 5b), indicating that plant productivity and soil extracellular enzymes play critical roles in TN accumulation following grazing exclusion. This is likely linked to the lack of atmospheric N inputs, because the atmospheric atmosphere N acquired by plants is shifted to the soil. Furthermore, a greater increase in soil C than in N in the 33-year succession likely caused the soil C:N ratio to increase (Fig. S1a), which likely increased soil N limitation. Grazing exclusion allows the plant diversity and vegetation community structures to recover; therefore, successive increases in plant productivity can ensure sufficient resources for microbial?mineralization?and N fixation. Thus, soil N accumulation may depend on the decomposition of roots and?mycorrhiza?by soil extracellular enzymes (especially N-acquiring enzymes) in soil?microbial communities?under N-limited consitions. As such, differences in soil microbial species appear to be strong determinants of soil N accumulation. However, our results showed that soil microbial properties, soil physical and chemistry properties, and vegetation characteristics could not illustrate the main varieties in TPS during grazing exclusion (Fig. 5c). Because of elevated water and nutrient availability (Table S2), this occurrence seems to be counter-intuitive, and the possible cause might be the leaching and exhaustion of TP. In addition, soil erosion also accelerates P loss on the Loess Plateau, and primary minerals become depleted as soil development proceeds, thus leading to decreases in the amount of P released by weathering. Strong competition between vegetation and?soil microbial communities?is also a key factor that leads to P insufficiency during grassland succession. Therefore, further studies are need to investigate the complex interactions between composition of microbial and plant communities with SOC, TN, and TP accumulation to grazing exclusion.?與SOCS相比,地下生物量和NAG對TNS的影響更大(圖5b),表明植物生產力和土壤胞外酶在禁牧后的全氮積累中起著關鍵作用。這可能與缺乏大氣氮輸入有關,因為植物獲得的大氣氮會轉移到土壤中。此外,在33年的演替過程中,土壤C的增幅超過N,這可能導致土壤C:N比值增加(圖S1a),這可能增加了土壤N限制。禁牧使植物多樣性和植被群落結構得以恢復;因此,連續(xù)提高植物生產力可以保證充足的資源用于微生物礦化和氮固定。因此,在氮有限的條件下,土壤氮的積累可能依賴于土壤微生物群落中土壤細胞外酶(尤其是氮獲取酶)對根和菌根的分解。因此,土壤微生物種類的差異似乎是土壤氮積累的強決定因素。然而,我們的結果表明,土壤微生物特性、土壤理化特性和植被特征并不能說明放牧封育期間TPS的主要變化(圖5c)。由于水分和養(yǎng)分利用率的提高(表S2),這種情況似乎與直覺不符,可能的原因可能是TP的淋溶和耗竭。此外,黃土高原的土壤侵蝕也加速了磷的流失,隨著土壤發(fā)育,原生礦物質逐漸枯竭,從而導致風化釋放的磷量減少。植被與土壤微生物群落之間的激烈競爭也是導致草地演替過程中磷不足的關鍵因素。因此,需要進一步研究微生物和植物群落組成與SOC、TN和TP積累對禁牧的復雜交互作用。
5.Conclusions
Grazing exclusion significantly increased SOCS at soil depths of 0–60cm, indicating that semiarid grasslands with fencing management can act as sinks for C sequestration. Grazing exclusion significantly enhanced TNS, but decreased TPS in the 0–40?cm soil layer. However, there were no significant differences in the TNS and TPS in the 40–60?cm soil layer during the 33?years following grazing exclusion. Furthermore, grazing exclusion is more favorable for SOC and TN accumulations than TP accretion, indicating that SOCS and TNS were decoupled from TP stock. Grazing exclusion had more effects on TN and TP stocks in the?topsoil?than in the?subsoil. Our results showed that plant community characteristics, such as cover and productivity, played an important role in SOC accumulation. Moreover, soil extracellular enzymes (especially N-acquiring enzymes) in?soil microbial communities?were found to play a key role in shaping soil N accumulation, whereas no factors were found to control variations in TPS following grazing exclusion. Overall, our findings are crucial for assessing the grazing and fencing measures linked to SOC, TN, and TP storages, and for the optimal recovery of degraded grasslands in semiarid areas.?禁牧顯著提高了0 ~ 60 cm土層SOCS,表明圍封管理的半干旱草地可作為碳固存的匯。禁牧顯著提高了0 ~ 40 cm土層TNS,但降低了TPS。而禁牧后33年間,40 ~ 60 cm土層TNS和TPS均無顯著差異。此外,放牧排斥比TP積累更有利于SOC和TN的積累,表明SOCS和TNS與TP存量脫鉤。放牧排斥對表層土壤TN和TP儲量的影響大于對底層土壤。結果表明:覆蓋度、生產力等植物群落特征在土壤有機碳積累中發(fā)揮重要作用。此外,研究發(fā)現(xiàn)土壤微生物群落中的土壤細胞外酶(尤其是氮獲取酶)對土壤氮積累起關鍵作用,而沒有發(fā)現(xiàn)放牧后土壤微生物TPS的變化。綜上所述,本研究結果對評估半干旱區(qū)退化草地的放牧和封育措施與SOC、TN和TP儲量的關系,以及對退化草地的最佳恢復具有重要意義。